1. INTRODUCTION
The Korea Pathfinder Lunar Orbiter (KPLO), also known as Danuri, achieved a major milestone on December 27, 2022 (UTC), when it entered its planned mission orbit to start exploring the Moon. After launch on August 4, 2022 (UTC), the KPLO was successfully inserted into a ballistic lunar transfer (BLT) trajectory and began a 4.5-month cruise to the Moon (Song et al. 2023a). The KPLO flight dynamics (FD) team monitored the spacecraft’s trajectory every business day and decided to perform four trajectory correction maneuvers (TCMs) to correct errors during its flight (Bang et al. 2022a; Bae et al. 2023). During the lunar orbit acquisition (LOA) phase, significant adjustments were made to the trajectory plan, leading to execute three lunar orbit insertion (LOI) maneuvers instead of the originally planned five LOI maneuvers and one orbit trim maneuver (OTM) (Song et al. 2023b, 2023c). Despite these changes, all mission orbit requirements were successfully met and the KPLO began operating nominally in a 100 km lunar polar orbit. To ensure the KPLO remains in its designated mission orbit, regular orbit maintenance maneuvers (OMMs) were performed and are scheduled in the future (Hong et al. 2023).
Space exploration missions inherently involve numerous expected and unexpected challenges. Since the KPLO mission is Korea’s first space mission outside Earth’s orbit and the BLT trajectory is challenging for beginners in space exploration, the KPLO FD team made a significant effort in preparing FD operations for both nominal and off-nominal situations. The trajectory design system and the FD subsystem were developed separately to achieve a flexible FD operation while reflecting the characteristics of BLT trajectory (Song et al. 2021; Bang et al. 2022b). Both systems were tested and validated through numerous simulations and rehearsals using realistic blind datasets (Song et al. 2022). Furthermore, cooperation with other entities was also prepared. Interfaces with National Aeronautics and Space Administration (NASA)’s Deep Space Network were established to facilitate the gathering of tracking data, as well as spacecraft’s telemetry and command (Song et al. 2023d). Collaborative efforts between Korea Aerospace Research Institute (KARI) FD team and NASA Johnson Space Center Flight Operations Directorate were made to compare orbit determination (OD) and maneuver planning (MP) solutions. A number of test and joint rehearsals were conducted to validate all operational concept, procedures, and timelines (Song et al. 2023e).
Preparing contingency trajectory operation is essential for the success of space exploration mission. In many previous lunar missions, a number of studies have been conducted on the recovery trajectories for off-nominal situations such as trans-lunar injection failure and LOI maneuver failure (Lozier et al. 1998; Beckman 2007; Kawakatsu et al. 2007; Genova 2014; Liu et al. 2015; Harpold et al. 2023). For the KPLO mission, LOI-related contingencies were analyzed under the phasing-loop transfer strategy, which was the strategy before adopting the BLT trajectory (Bae et al. 2017; Song et al. 2017). After the confirmation of the BLT trajectory, a comprehensive contingency analysis was performed across all mission phases, leading to the development of mitigation plans for each trajectory contingency case (West et al. 2022; Bang et al. 2023). Note that most studies have primarily focused on how to mitigate specific contingencies and design recovery trajectories. Unlike previous references, this paper encompasses the entire contingency preparation process, from identifying and prioritizing trajectory contingencies to developing mitigation plans and operational procedures. This thoroughly prepared contingency trajectory operation plan enabled KPLO to successfully overcome not only minor contingencies during actual operations but also significant and sudden changes in the trajectory plan during the LOA phase through flexible operation.
The remainder of this paper is structured as follows. Section 2 provides an overview of KPLO’s nominal trajectory and mission orbit. Section 3 presents the methodologies and results related to the identification and prioritization of trajectory contingencies. Preparation of recovery plans and operational procedures for contingency trajectory operation are introduced in Section 4. Finally, Section 5 provides the conclusions.
2. TRAJECTORY AND MISSION ORBIT OVERVIEW
The KPLO utilized a BLT trajectory to reach the Moon. The trajectory was designed to ensure consistent lunar arrival conditions, regardless of the launch date. Prior to launch, the KPLO FD team prepared reference trajectories corresponding to each launch date within the 7-day launch period. During the trans-lunar cruise (TLC) phase, a total of nine TCMs, namely TCM1 through TCM9, were scheduled to correct any errors during the flight. TCM1 serves the dual purpose of addressing launch errors and testing the orbit maneuver thruster (OMT), which is a newly developed propulsion system. TCM3 is the only deterministic maneuver with a non-zero magnitude and direction for the purpose of manifold transition. The others are statistical maneuvers that can be performed when the spacecraft is expected to fail to achieve the desired lunar arrival conditions. Fig. 1 illustrates the nominal KPLO trajectory for the August 4, 2022 launch case, including the locations where TCMs are scheduled.
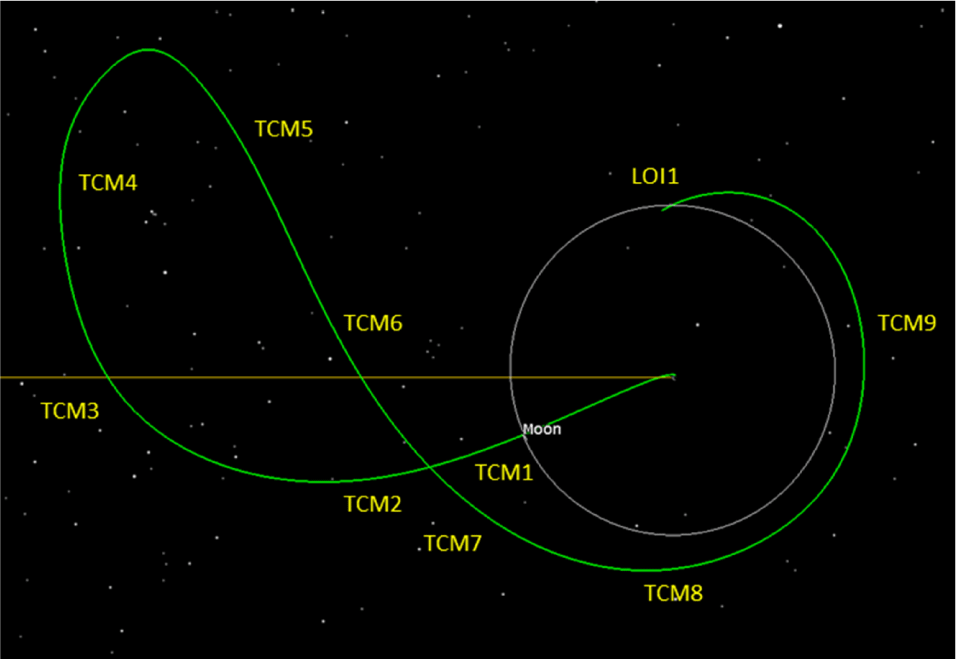
The LOA phase originally included five LOI maneuvers and one OTM, namely LOI1 through LOI5 and OTM1, respectively. Each LOI maneuver is an anti-velocity burn executed at the perilune to reduce the orbit period. OTM1 consists of a pair of maneuvers designed to strictly match a 100 × 100 km circular orbit at the end of the LOA phase. The commissioning phase was scheduled after the LOA phase to check the operability of science payloads. One additional OTM, OTM2, was planned during the commissioning phase to achieve mission orbit requirements: 100 ± 30 km of lunar altitude and 90 ± 0.25 deg of mean inclination during the 1-year mission period. After the beginning of the nominal mission phase, regular OMMs were planned to maintain the KPLO’s orbit. Each OMM is composed of a pair of maneuvers that adjust eccentricity and argument of periapsis simultaneously.
During the actual operation of KPLO, especially in the LOA phase, the trajectory plan has been significantly revised regarding many real-world operational constraints (Song et al. 2023c). Note that the results in this paper are based on the reference trajectory generated prior to launch.
3. TRAJECTORY CONTINGENCY
The first step in preparing for contingency trajectory operations is to identify all off-nominal situations that could impact the KPLO’s trajectory. Table 1 provides a summary of the predicted performance of the launch vehicle and KPLO thrusters, namely OMT and attitude control thruster (ACT), which were received prior to launch. Based on these values, the KPLO FD team conducted dispersion analysis and prepared the delta-V budget to account for expected errors during launch and maneuver executions.
Launch vehicle accuracy (3σ) | |
C3 | 0.15 km2/s2 |
RAV | 0.2° |
DAV | 0.2° |
Maneuver magnitude accuracy (3σ) | |
OMT | 4% |
ACT | 4% |
Maneuver pointing accuracy (3σ) | |
OMT | 1.27° |
ACT | 2.5° |
A contingency can be simply considered as any situation outside of the expected performance and the established delta-V budget. During the launch phase, a total of six contingencies were identified as presented in Table 2. Given the instantaneous launch window for the KPLO trajectory on each launch date, the launch time error can impact the trans-lunar trajectory insertion performance. Low C3 and high C3 energy cases were separately considered. If the launch vehicle fails to produce sufficient C3 energy, there is a risk that the KPLO will return to Earth’s orbit. On the other hand, if the launch vehicle generates excessive C3 energy, the KPLO may fly towards the Sun instead of the Sun-Earth L1 point. Contingencies in KPLO’s trajectory also arise when errors in right ascension of the injection orbit apoapsis vector (RAV) and declination of the injection orbit apoapsis vector (DAV) exceed the expected boundaries. Additionally, the risk of collision with any satellites orbiting in Earth’s orbit was considered as a potential contingency.
L, likelihood; C, consequence; TCM, trajectory correction maneuver; LOI, lunar orbit insertion; OTM, orbit trim maneuver; OMM, orbit maintenance maneuver; C3, launch injection energy per unit mass; RAV, right ascension of the injection orbit apoapsis vector; DAV, declination of the injection orbit apoapsis vector; Col, collision; Az, azimuth; El, elevation.
In the TLC phase, contingencies can be categorized into two cases: those occurring before and after TCM execution. When planning a TCM, not only the upcoming maneuver but also all remaining TCMs until lunar arrival are comprehensively considered to predict the remaining fuel after the TLC phase. If the expected fuel consumption exceeds the assigned fuel budget, there is a risk of violating the mission orbit requirements. One critical contingency associated with TCMs is the failure to execute a planned TCM. Since TCM3 is an essential maneuver for lunar approach, missing its execution could result in a lunar approach failure. Execution failures in other statistical TCMs and unexpected errors in magnitude, attitude, and burn time could lead to off-nominal trajectories.
Before the LOA phase, contingencies related to errors in lunar arrival conditions following the final TCM, TCM9, were identified. These errors include lunar arrival epoch, altitude, and inclination, all of which can impact the trajectory during the LOA phase. Similar to TCM planning, when planning a LOI maneuver, all remaining maneuvers until the end of the LOA phase are planned together to predict fuel consumption. A lack of remaining fuel could lead to a failure in achieving the mission orbit requirements. The conjunction risk with other lunar orbiters including the Lunar Reconnaissance Orbiter (LRO) and Chandrayaan-2 was considered as a contingency case that requires special operations. The first LOI maneuver, LOI1, is responsible for capturing the KPLO in lunar orbit. Failure to execute LOI1 or a significant partial burn during LOI1 could result in a failure in lunar capture. Execution errors that exceed the predicted values in magnitude, attitude, and burn time during the LOA phase could lead to off-nominal orbits.
The purposes of OTMs and OMMs are to ensure that the KPLO remains within the mission orbit requirements: an altitude of 100 ± 30 km and a mean inclination of 90 ± 0.25 deg during the 1-year mission period. Failures in executing these maneuvers or unexpected execution errors in magnitude, attitude, and burn time may result in a violation of the mission orbit requirements.
After identifying trajectory contingencies, the KPLO FD team prioritized them to efficiently prepare recovery plans. The prioritization was based on evaluating likelihood and consequence, which are widely used method in risk analysis. Likelihood refers to the probability of a contingency case occurring and was scored on a 5-point scale in this study: 1) least likely, 2) unlikely, 3) moderate, 4) likely, and 5) most likely to happen. Consequence represents the impact when a contingency occurs and was also assessed on a 5-point scale: 1) minor impacts requiring additional analysis, 2) mild impacts that can lead to a change in mission timeline, 3) moderate impact that can lead to a reduction of mission lifetime, 4) significant impacts that can lead to the loss of one or more science objectives, and 5) severe impacts that can lead to the loss of mission. Each team member qualitatively evaluated the likelihood and the consequence for each contingency case, and the average values were used for prioritization, as presented in Table 2. In addition, the complexity of preparing recovery plan for each contingency was also evaluated to consider the practical timeline and ensure completion of the preparation process before launch.
All contingencies were categorized into three priority groups based on the multiplication of likelihood and consequence (L × C) scores: 1) 15–25, 2) 8–14, and 3) 1–7. Priority 1 group includes three contingencies associated with LOI1. Since the LOI1 maneuver is the most critical maneuver for lunar capture, any failure or partial burn during the maneuver could directly result in a loss of mission. Therefore, a mitigation plan for these contingencies should be prepared as a top priority based on in-depth analysis results. Priority 2 group consists of four categories: low C3 energy during launch, execution failure of TCM3, errors in lunar arrival, and azimuth pointing errors during LOA phase. These contingencies have non-negligible likelihood and consequence that could lead to a loss of science objectives or a reduction of mission lifetime. Azimuth-directed pointing errors are more significant than elevation-directed errors because the KPLO has a strict inclination requirement to observe the Moon’s south pole. Other contingencies were classified as Priority 3, which have a low probability or minor impacts on KPLO’s trajectory.
4. CONTINGENCY TRAJECTORY OPERATION PLAN
The KPLO FD team developed mitigation plans for each trajectory contingency based on their assessed priorities. High-priority and complex contingencies were analyzed before other cases. For each contingency case, a sensitivity analysis was conducted to quantitatively assess the resulting trajectories depending on the severity of the contingency. In general, there are several viable options for addressing trajectory contingencies, such as rescheduling a maneuver, adding one or more recovery maneuvers to follow the reference trajectory, or revising the reference trajectory itself. The KPLO FD team determined the most preferable and effective option for each case and prepared corresponding mitigation strategies.
Fig. 2 illustrates the off-nominal trajectory and the recovery trajectory for the case of LOI1 maneuver execution failure. In the event of a failed LOI1 execution, the KPLO would arrive at the next perilune with a significantly different altitude and inclination than the desired conditions, ultimately escaping the Moon as depicted by the red trajectory in Fig. 2. Simply adding a recovery LOI (rLOI) maneuver at the next perilune, as a substitute for the original LOI1, requires enormous delta-V for inclination correction. To reduce the required delta-V, a recovery TCM (rTCM) is also included ahead of the rLOI to achieve the desired altitude and inclination at the perilune. The location of the rTCM is strategically determined at the apolune to ensure sufficient time for OD and MP for both maneuvers. Consequently, the KPLO can be captured into the desired lunar orbit with an additional delta-V of approximately 85.75 m/s. Similar to this case, recovery plans were prepared for all identified trajectory contingencies based on the results of contingency analysis and practical considerations, including operational timelines.
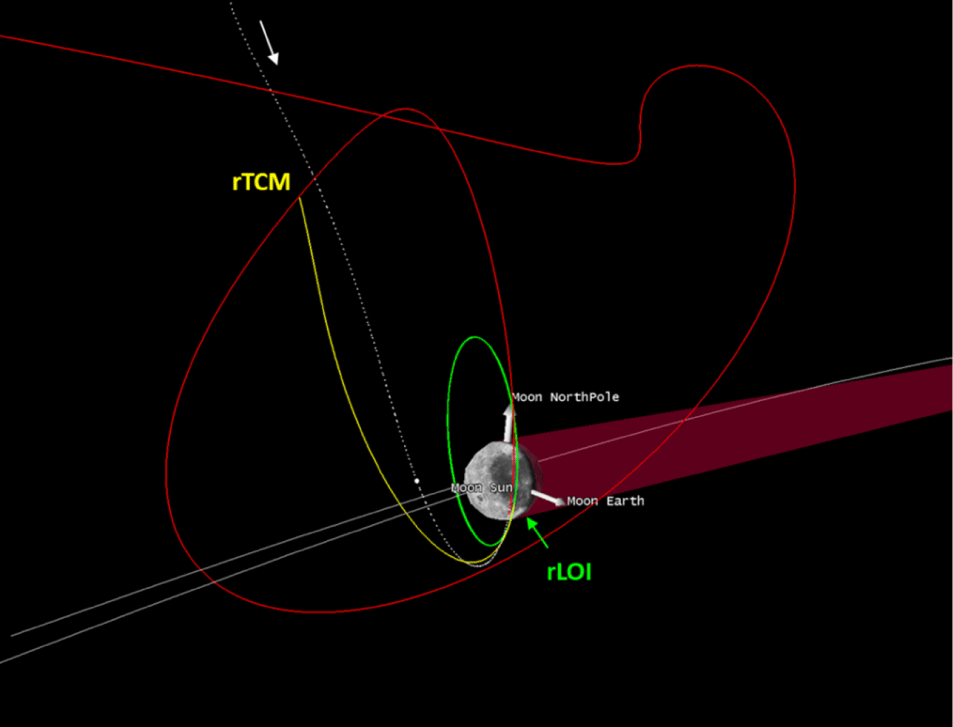
To ensure smooth and immediate trajectory operations in the event of trajectory contingencies, the KPLO FD team developed a document titled “Trajectory Contingency Playbook” (Bang 2022c). This document provides not only the list of trajectory contingencies identified in Section 3, but also detailed operational procedures based on the prepared recovery plans. Fig. 3 outlines the workflow for contingency trajectory operations. When an off-nominal situation occurs, FD operators can find the corresponding case ID and section numbers in the contingency list. Each section contains checklists or guidelines that FD operators can intuitively follow. As a brief example, if the LOI1 maneuver fails to execute as planned, FD operators are instructed to: 1) replace the nominal template scenario with the contingency scenario, which includes rTCM and rLOI, 2) check the feasibility of executing rTCM on time, 3) check that there is enough time to plan a maneuver between all maneuvers during the LOA phase, and 4) determine a target inclination value for rTCM that meets the mean inclination requirements as per step-by-step instructions. The procedure was validated through simulations performed prior to launch and remains revisable to address any unexpected issues that may arise during the mission.
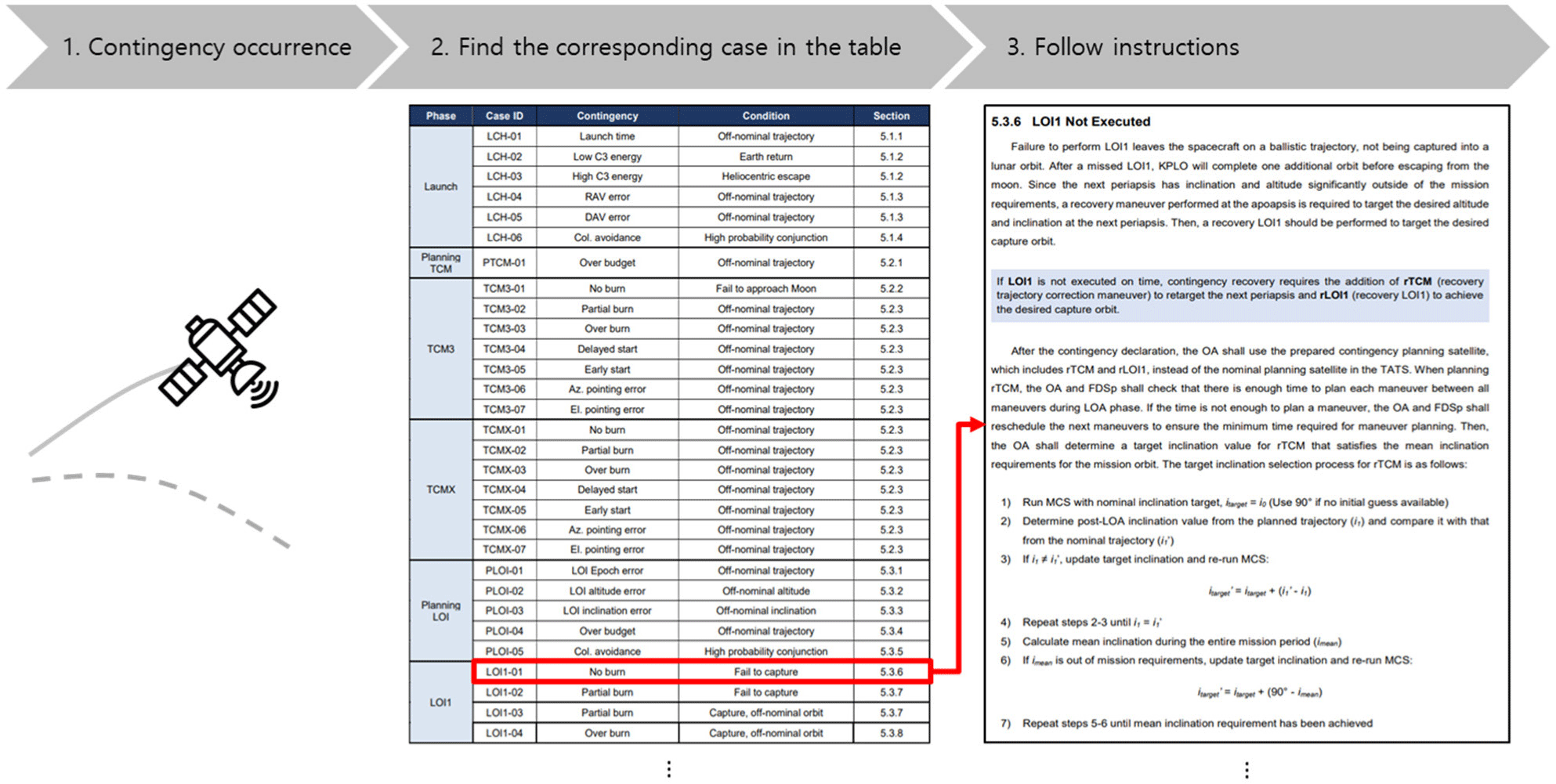
5. CONCLUSIONS
This paper provides a comprehensive overview of the process of preparing contingency trajectory operations for the KPLO. To ensure the success of the mission and to account for potential off-nominal situations, the KPLO FD team conducted a thorough analysis of trajectory-related contingencies across all mission phases, including launch, TLC, LOA, commissioning, and nominal mission. The team’s initial step involved identifying a wide range of potential trajectory contingencies, drawing from the expected performance of the launch vehicle and KPLO thrusters. The application of likelihood and consequence assessment allowed the team to prioritize trajectory contingencies effectively. High-priority cases, particularly those related to LOI1, were addressed with in-depth analysis due to their critical impact on the mission’s success. The paper outlines the development of mitigation plans and recovery strategies for each trajectory contingency. These strategies encompass various options, such as rescheduling maneuvers, adding recovery maneuvers, or modifying the reference trajectory. To ensure swift and well-coordinated trajectory operations in the event of contingencies, the KPLO FD team developed a document for contingency trajectory operations. This document contains detailed operational procedures, checklists, and guidelines for FD operators, facilitating a systematic approach to address specific contingency scenarios.
In summary, this study offers valuable insights for FD engineers and mission planners engaged in space exploration missions, emphasizing the importance of a proactive approach to contingency planning. The preparation process, from identification and prioritization of trajectory contingencies to the development of mitigation plans and operational procedures, serves as a comprehensive guide for ensuring the success of future space exploration missions. Fortunately, the KPLO mission encountered no serious contingencies. Two minor contingencies were effectively addressed using prepared plans: the over burn during TCM5 was corrected with TCM6, and the conjunction risk with Chandrayaan-2 was mitigated through a collision avoidance maneuver. Furthermore, the experience and know-how gained from the preparation process allowed flexible operations, even when significant adjustments were required to the trajectory plan during the LOA phase. The KPLO mission's achievements provide an excellent example of how diligent preparation can lead to mission success even in the face of unexpected challenges.