1. INTRODUCTION
On August 4, 2022 (UTC), the successful launch of the Korea Pathfinder Lunar Orbiter (KPLO), the Republic of Korea’s first lunar exploration spacecraft, was achieved using a SpaceX Falcon 9 rocket. As of July 2023, all systems of the KPLO are operating as expected (Bae et al. 2023; Hong et al. 2023; Jeon & Cho 2023; Kim et al. 2023; Song et al. 2023). The initial trajectory design for the lunar transfer phase of the KPLO employed a commonly used approach called the 3.5 phasing loop orbit (Choi et al. 2017), which is often utilized by entities undertaking their first lunar missions. The development of KPLO appeared to proceed smoothly, as Korea had extensive experience in the development and operation of low Earth orbit and geostationary satellites. However, the KPLO program office encountered a challenge in dealing with the issue of mass increases of the KPLO. Notably, the program office was faced with this issue nearly at the completion stages of hardware development, including the fuel tanks. This presented significant challenges in initiating a redesign of the hardware, particularly the fuel tanks, starting from scratch.
Due to the mass increases, the KPLO required more onboard fuel and consequently, the primary mission objective was not feasible to be sustained. The primary objective of the KPLO mission was to maintain a circular polar orbit around the Moon with an altitude of 100 km and an inclination of 90 degrees for a duration of one year. A proposal was made to modify the mission orbit to a polar elliptical orbit to mitigate fuel shortage issues which had a periselene altitude of 100 km and an aposelene altitude of 300 km (Park 2019). However, the newly proposed mission orbit raised concerns because it did not take into account the operational needs of KPLO’s scientific payloads. This led to opposition as it was not discussed with the KPLO science community beforehand and did not meet the minimum scientific objectives of the mission. Instead of altering the target mission orbit, a decision was made to change the lunar transfer method from the originally planned 3.5 phasing loop orbit to the ballistic lunar transfer (BLT) trajectory, which was recommended by the National Aeronautics and Space Administration (NASA). The NASA became a joint partner in the KPLO program, as part of the agreement made in 2016 between the Korea Aerospace Research Institute (KARI) and NASA. In exchange for onboarding the U.S payload, the ShadowCam, NASA agreed to provide the deep space network (DSN) service and independent verification and validation (IV&V) of KPLO navigation (Sofge & Kim 2016).
Although the BLT trajectory, known for its greater fuel savings over the 3.5 phasing loop transfer, was chosen, it did not fully resolve all the issues. This was primarily due to the fact that the KPLO bus system had been designed and developed based on the assumption of the 3.5 phasing loop orbit. Additionally, at the time of the KPLO launch, the Gravity Recovery and Interior Laboratory (GRAIL) mission was the only other mission that intentionally planned to utilize the BLT trajectory (Hatch et al. 2010), indicating that the KPLO team lacks sufficient past missions to reference. Considering that the KPLO mission was Korea’s first lunar exploration, this background posed even more challenges for KARI. Thorough analyses were conducted by all KPLO engineers to assess the compatibility of the KPLO system with the BLT trajectory. In particular, the flight dynamics (FD) team focused on an in-depth analysis to evaluate the impact of KPLO’s propulsion and attitude control systems’ errors on the overall Delta-V budget. During this analysis, it was confirmed that the accuracy of the launch vehicle’s (LV’s) trajectory insertion became even more critical when adapting the BLT trajectory to the KPLO mission, due to the sensitivities posed by FD upon BLT. Any significant BLT trajectory insertion error would result in a dramatic increase in onboard fuel consumption, requiring prompt and efficient execution of subsequent correction maneuvers. These factors truly influenced the achievement of the overall mission goal and the feasibility of an extended mission.
This paper presents the analysis results of KPLO’s trajectory insertion performance conducted using real flight operational data, and highlights the significant contribution it made to achieve the ultimate mission goal. The current work discusses the conditions established at the target interface point (TIP) to ensure a successful insertion into the BLT trajectory. Also includes details of the launch performance evaluation procedure conducted during real flight operation. The accurate insertion of KPLO into the target BLT trajectory played a critical role in facilitating the smooth operation of the remaining journey to the Moon after LV separation. Moreover, it ensured a larger fuel reserve allocated for margins, thereby increasing the potential for an extended mission. The analysis results and the procedure based on actual flight data in this paper will serve as a valuable resource for examining the performance of LVs in future space exploration missions planned in Korea. The paper is organized as follows: Section 2 provides an overview of the BLT trajectory for the KPLO mission, emphasizing the launch sequence of the SpaceX Falcon 9 and the allowable target trajectory insertion boundaries given for mission success. In Section 3, a detailed analysis procedure and the corresponding results of the target trajectory insertion performance, conducted by the KPLO FD team immediately after KPLO separation, are presented. Finally, Section 4 concludes the paper.
2. KOREA PATHFINDER LUNAR ORBITER (KPLO) LUNAR TRANSFER TRAJECTORY
In order to design the reference trajectory for the trans lunar cruise (TLC) phase using BLT, the KPLO FD team closely collaborated with SpaceX, the launch provider. Unlike other launch trajectories, the BLT trajectory does not have a duration-based launch window; instead, it has an instantaneous launch window. Therefore, obtaining precise launch information from the launch provider is crucial for designing the reference trajectory. The reference trajectory for KPLO’s TLC phase was designed by considering specific launch conditions received from the launch provider, as well as the lunar arrival conditions for KPLO.
The parameters obtained from the launch provider that were crucial for designing the reference trajectory included the precise launch site location, the Second stage engine cut off 1 (SECO-1) state vector in the Earth-centered, Earth-fixed (ECEF) frame, the preferred coasting option, and constraints on the argument of periapsis to ensure reliable ground tracking during the launch phase. Among these parameters, the SECO-1 state vector in the ECEF frame played the most important role in designing the reference trajectory as it was directly used for the initial states to derive the target BLT trajectory insertion parameters at TIP. For the lunar arrival conditions, the time of arrival and the six orbital elements that describe the lunar orbit at the arrival point were considered. Among the lunar arrival conditions, the time of arrival at the Moon can be determined by taking into account the duration of the lunar orbit acquisition (LOA) phase, the commissioning period, and the visibility with ground stations. The lunar orbit’s right ascension of the ascending node at the arrival point was determined by analyzing the lunar solstice condition, which was one of the major scientific requirements driven by NASA’s payload, the ShadowCam.
Using the above Earth departure and lunar arrival constraints, the KPLO FD team underwent numerous trials and errors to finalize the reference BLT trajectory suitable for the KPLO. Considering its highly sensitive dynamics, a combination of forward and backward numerical targeting methods was employed to design the reference BLT trajectory. Careful consideration was given to select appropriate perturbing forces, suitable targeting parameters, and associated tolerances to ensure the integrity of the BLT trajectory’s dynamic model while achieving fast simulation time. Once the reference BLT trajectory was determined for a specific date, it was extended to obtain reference trajectories for various launch period spans. Throughout this process, multiple iterations between the KPLO FD team and SpaceX team were made to ensure that the launch conditions for the generated launch periods aligned with the flight requirements of the LV. The KPLO FD team provided and updated the launch provider with the reference trajectory sets several times, covering different launch period spans. Approximately 10 days prior to launch, a final candidate launch period from Aug. 2nd to Aug. 8th, 2022, was delivered to the launch provider. Fig. 1 illustrates the KPLO BLT trajectory for a launch period of approximately 30 days in the Sun-Earth rotating frame, targeting a Moon arrival on Dec. 16th, 2022. The time of flight (TOF) varies depending on the launch date, with an approximate TOF of 137 days for the opening of the launch period on Jul. 31st and approximately 109 days for the closing of the launch period on Aug. 29th. For more detailed results regarding the reference trajectory design of KPLO’s TLC phase, readers may refer to the works done by Lee et al. (2020a, 2020b, 2020c).
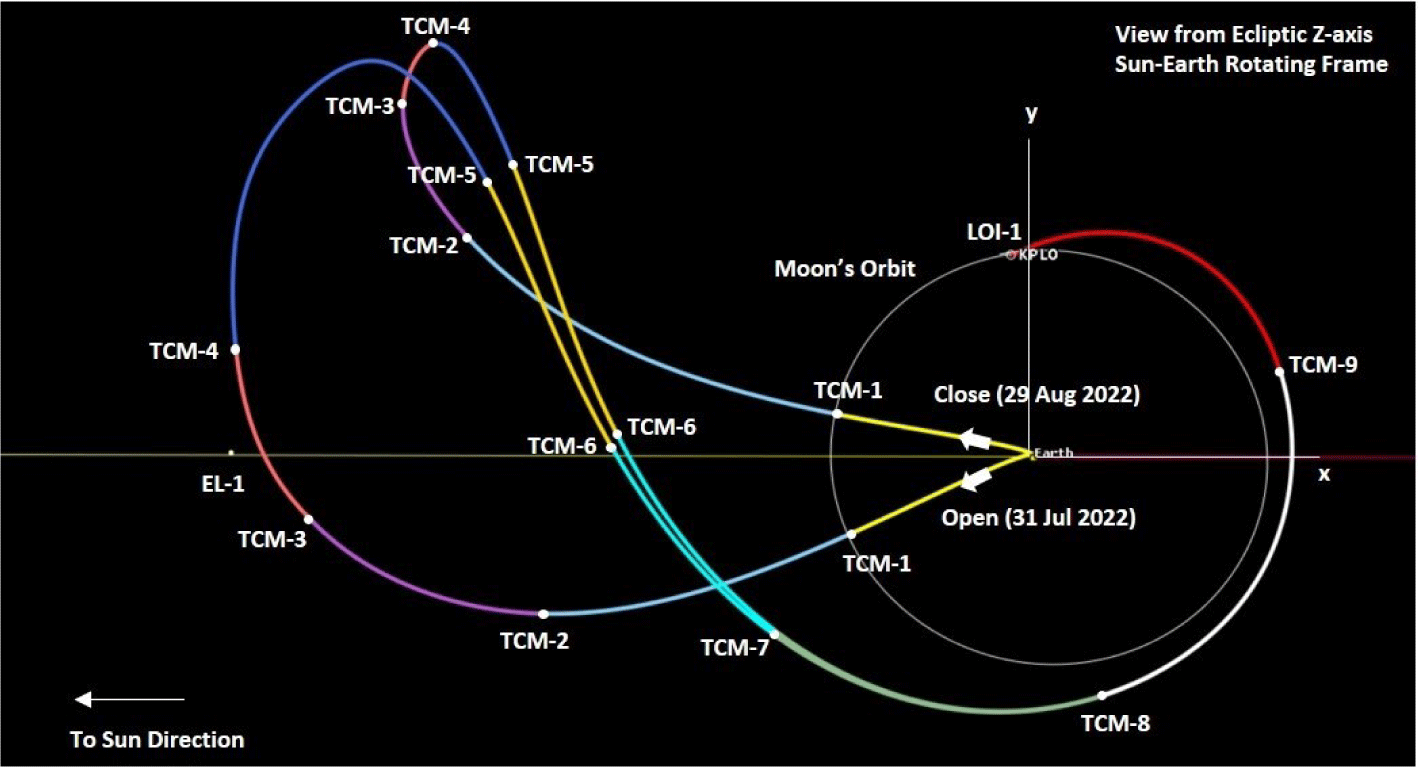
The KPLO mission was designated as flight #169 of the Falcon 9 LV (F9-169), and F9-169 involved two burns to deploy the KPLO into the BLT trajectory. Table 1 illustrates the launch sequence along with the corresponding abbreviated mission timeline for the launch date of Aug. 4th, 2022. It is important to note that the timeline is subject to slight changes depending on the actual launch date and any final updates to the reference trajectory.
The Falcon 9 rocket assigned to the KPLO mission had already completed five Stage 1 launches, and the fairing had been used three times. As shown in Table 1, approximately 2.52 minutes after liftoff, the main engine cut off (MECO) occurred, followed by the separation of the fairing about 38 seconds later. The target orbit insertion for the LV itself was defined as SECO-2 plus 10 seconds. Approximately 40 minutes after liftoff, near SECO-2 + 5 minutes, the KPLO spacecraft would be separated from the LV. The TIP, which represents the reference point for calculating the KPLO’s BLT trajectory insertion parameters, was defined as SECO-2 + 10 minutes. As described earlier, the KPLO FD team designed the BLT reference trajectory and provided the launch provider with the target insertion parameters at TIP, which were generated based on the designed reference trajectory. The provided parameters included the TIP epoch, launch injection energy per unit mass (C3), right ascension of the injection orbit apoapsis vector (RAV), and declination of the injection orbit apoapsis vector (DAV). Table 2 shows the values of TIP Epoch, C3, RAV, and DAV at TIP corresponding to the final candidate launch period of one week.
In Table 2, the parameters C3, RAV, and DAV are defined in the Earth Mean Equator and Equinox of Epoch J2000 (EME2000) frame, where J2000 represents JD 2451545.0 barycentric dynamical time (TDB), corresponding to January 1, 2000, 12:00:00.000 TDB. Within this reference frame, the x-axis aligns with the Vernal equinox, the z-axis is perpendicular to the mean equator at the J2000 epoch, and the y-axis is defined as the cross product of the z-axis and the x-axis. RAV is measured from the x-axis to the projected vector of the apoapsis vector onto the reference plane, and DAV is measured from the reference plane to the apoapsis vector in the vertical direction. Additionally, it is important to note that all targets employ a short coast, meaning that the true anomaly in the parking orbit is limited to less than 180 degrees. Allowable dispersions for each target insertion parameter were given as ± 0.15 km2/s2 for C3 and ± 0.2 degrees for both RAV and DAV, respectively (Presti 2022).
3. INSERTION PERFORMANCE ANALYSIS
KPLO successfully lifted off on Aug. 4th at 23:08:48 UTC within the final candidate launch period provided to the launch provider. After entering the BLT trajectory, KPLO planned nine trajectory correction maneuvers (TCMs) prior to lunar arrival but executed only four of them (TCM-1, TCM-3, TCM-5, and TCM-6). Fig. 2 depicts the lift-off of KPLO on Aug. 4th and the corresponding BLT trajectory on that day in the Earth-centered, Sun-Earth rotating frame, specifically the ecliptic Z-axis projection view.
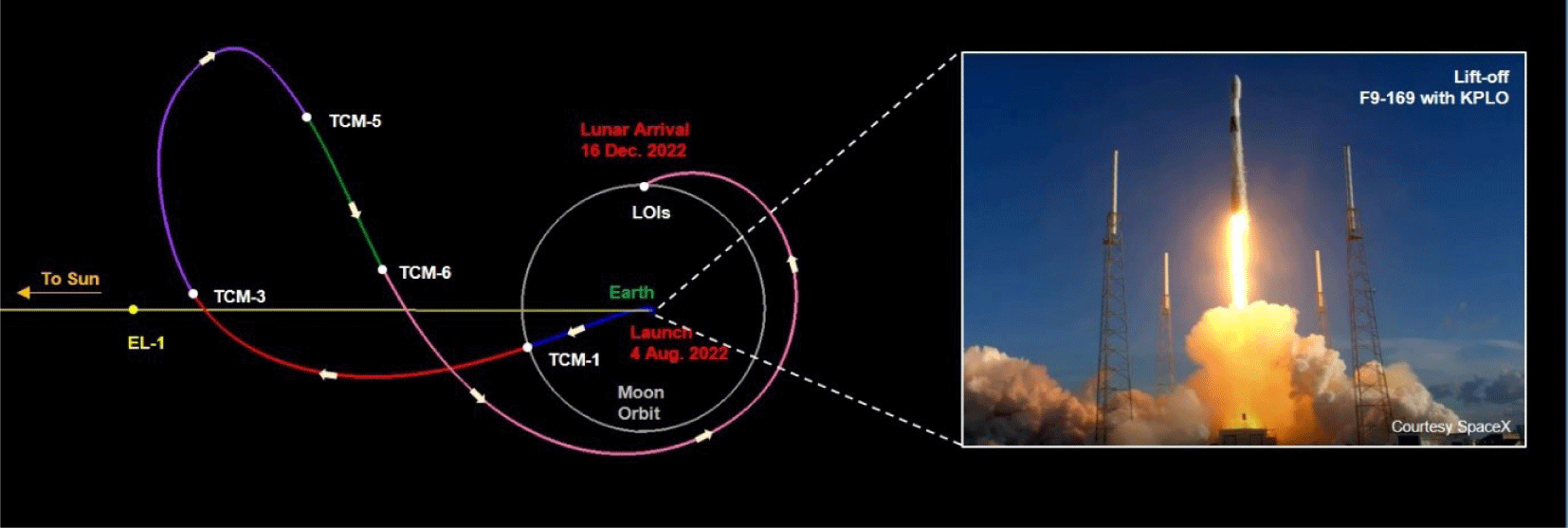
The analysis of the LV’s target trajectory insertion performance cannot be completed in a single iteration. It requires an initial analysis using the orbit parameter message (OPM) received from the launch provider right after KPLO separation, followed by multiple iterative analyses using the orbit determination (OD) solutions. Even though OPM can be very useful for a quick analysis of LV performance, subsequent analysis based on OD solutions is necessary. This is because the state uncertainties, especially in the accuracy of velocity knowledge, in the OPM may sometimes be degraded since the separation vector is the final state that are received for the state determination. Additionally, the states in the OPM do not include the effect of spacecraft separation velocity since the states within the OPM represent the upper stage rather than the separated spacecraft itself. This section presents the results and the process of analyzing the post-trajectory insertion performance based on the OPM and OD solutions.
An initial analysis of the target trajectory insertion performance was conducted based on the OPM provided by the launch provider. The OPM, a text file, contained LV separation information, including the liftoff time, current state time, mission elapsed time, position and velocity information in ECEF coordinates, and orbital elements. Prior to the KPLO launch, the KARI FD team collaborated with SpaceX team to format the OPM, and KARI implemented additional functions in the FD-related software to utilize this OPM as input for a quick analysis of LV performance. It was expected that the launch provider would deliver the OPM to KARI within 30 minutes after the targeted TIP epoch. However, surprisingly, the KARI FD team received the OPM only 31 seconds after the target TIP epoch. Upon receiving the OPM, a quick analysis of the target trajectory insertion performance was conducted. As the LV states in the OPM were prior to the TIP epoch, the FD team forward-predicted the states at the TIP epoch and examined the launch performance. The results revealed differences between the target and achieved TIP parameters, approximately 0.013 km2/s2 for C3, 0.080 degrees for RAV, and 0.042 degrees for DAV, respectively. These differences were found to be accurate, surpassing the expected values. Despite being quick analysis results, the FD team was able to prepare the following OD and TCM-1 analysis with greater confidence based on these findings.
Following the initial analysis of the LV insertion performance using the OPM, additional analyses were conducted using the OD solution in a total of three iterations. The first analysis was based on the OD solution using tracking data up to 8 hours after launch. The second and third analyses utilized tracking data up to 25 hours and 34 hours after launch, respectively. KPLO primarily utilized NASA’s DSN during the TLC phase for flight operations, and the DSN provided radio metric tracking data in the DSN tracking system data archival format (TRK-2-34) or tracking data message (TDM) format.
The FD team conducted continuous OD in near real-time, utilizing tracking measurements received from the DSN starting right after KPLO separation. During the approximately 2-hour period following separation, OD was performed using only DSN TCP data. This was necessary due to the bus system’s initial checkout activities during this timeframe. After the initial 2 hours, DSN ranging data was received, and OD was successfully conducted using both DSN ranging and TCP Doppler data. The FD team continuously derived OD solutions while simultaneously monitoring the stability of the solutions and the resulting launch performance. The stability of the OD solutions and launch performance was crucial as it directly affected the quality of the planned TCM-1 maneuver scheduled 48 hours after launch. Therefore, it was a critical factor that required continuous monitoring. Table 3 presents the estimated LV performance using tracking data from 8, 25, and 34 hours. It also displays the OD uncertainty (3-sigma) and the associated results of the planned TCM-1 for each case. Here, note that the OD uncertainties shown in Table 3 are the 3-dimensional (3D) root mean square (RMS) values of each tracking arc utilized—8, 25, and 34 hours.
To determine the LV’s target trajectory insertion performance using OD solutions, the states were backward predicted, unlike analysis conducted with OPM, from each OD epoch to the TIP epoch. As shown in Table 3, the target parameters of C3, RAV, and DAV gradually converged to specific values as more tracking data were incorporated into the OD solutions. Simultaneously, the planned magnitude of TCM-1 Delta-V also converged to approximately 3.080 m/s. This convergence was accompanied by a decrease in OD uncertainty, particularly in velocity uncertainty, which was approximately 0.95 cm/s.
As shown in Table 3, the LV accurately inserted the KPLO into the target BLT trajectory. The LV achieved the specified target TIP parameters (C3, RAV, and DAV) with an accuracy within the original allowable dispersion boundaries by more than two orders of magnitude. This high level of accuracy enabled the successful execution of the TCM-1 maneuver without significant deviations from the original plan. The TCM-1 maneuver was one of the most critical maneuvers as it aimed to correct the LV separation error while conducting a health check burn simultaneously for the orbit maneuver thruster (OMT) for the first time before actively using it in orbit. In fact, a previous LV dispersion analysis indicated that a margin of approximately 60 m/s (3-sigma) was required for the TCM-1 burn (Hong et al. 2020). Based on OD solutions using 34 hours of tracking data, the final TCM-1 burn plan was uploaded to the KPLO with a Delta-V of 3.080 m/s. The actual Delta-V achieved during the TCM-1 burn was approximately 2.927 m/s, indicating a slight cold burn. As a result of the launch provider’s highly accurate insertion of the KPLO into the BLT, approximately 19 kg of fuel, which had been reserved as a margin for TCM-1, could be utilized for the science mission. Considering that KPLO requires approximately 24 kg of fuel to maintain its mission orbit around the Moon at an altitude of 100 ± 20 km for one year, the additional amount of secured fuel is definitely significant which cannot be ignored. This successful BLT insertion facilitated smooth operations not only during the remaining TLC phase but also during the LOA phase, enabling additional extended missions for the KPLO.
4. CONCLUSIONS
In this work, KPLO post trans-lunar trajectory insertion performance analysis results as well as procedure conducted during the actual flight are summarized. As with the changing KPLO’s lunar transfer methods from the 3.5 phasing loop orbit to the BLT trajectory, the importance of trajectory insertion performance has significantly increased. This is because the post trajectory insertion accuracy directly affects the consumption of onboard fuel, which in turn impacts the successful achievement of overall mission objectives and further the possibility of extended missions. To ensure a successful insertion into the BLT trajectory, the KPLO FD team closely collaborated with SpaceX team, the launch provider. The FD team firstly designed the reference BLT trajectory specialized for KPLO, and delivered the launch provider the target insertion parameters for each potential launch date, including the TIP epoch, C3, RAV, and DAV values.
Since the separation of KPLO from the LV, the FD team has devoted significant efforts to analyzing the post-trajectory insertion performance and understanding its significance in relation to the successful achievement of the final mission goal. Initially, a quick analysis was conducted using the OPM provided by the launch provider. Subsequently, a more thorough analysis was carried out using OD solutions. By utilizing DSN tracking measurements collected up to 8, 25, and 35 hours after launch, the post-trajectory insertion performance was estimated iteratively, and corresponding TCM-1 burn plans were also established for each of the three cases. As a result, achieved target insertion parameter of C3, RAV, and DAV at TIP converged as more tracking data were incorporated reveling that very accurate achievement of target trajectory insertion made by the LV. It was confirmed that all trajectory insertion parameters successfully achieved their target values at the TIP epoch, with an error level of approximately 0.007 km2/s2 for C3, 0.079 degrees for RAV, and 0.042 degrees for DAV. The highly successful insertion of KPLO into the BLT trajectory allowed for precise execution of the TCM-1 maneuver with minimal deviations from the original plan. As a result, approximately 60 m/s of Delta-V margin, equivalent to about 19 kg of onboard fuel allocated for the TCM-1 burn, could be utilized for the science mission operations. The amount of fuel saved is slightly less than to the fuel required for KPLO to maintain an altitude range of 100 ± 20 km at the Moon for approximately one year. This successful BLT insertion greatly reduced the operational burden for the operators and facilitated the smooth progression of subsequent mission phases, enabling the possibility of extended missions for KPLO.