1. INTRODUCTION
Due to Korea Pathfinder Lunar Orbiter (KPLO)’s successful insertion into the lunar orbit, Korea became the world’s 7th lunar exploration country. On Aug. 4, 2022, 23:08:48 (UTC), the KPLO, also known as Danuri, was launched using SpaceX Falcon 9 launch vehicle. The KPLO’s successful lunar orbit insertion (LOI) was confirmed on Dec. 27, 2022, and currently, KPLO is orbiting the Moon having 100 ± 20 km altitude above the lunar surface with 90 deg inclination. Recently, the KPLO’s bus system itself, as well as the onboard payloads’ health check, was completed through the commissioning phase. In addition, payload calibration activities were also conducted, and now the KPLO is performing the mission operation around the Moon. To date, all KPLO systems are operating nominally.
It is well known that the efficient utilization of ground antenna is critical for the spacecraft’s telemetry receiving and commanding. Moreover, receiving well-calibrated tracking measurements is another crucial factor for successful flight dynamics (FD) operation. The KPLO utilized the National Aeronautics and Space Administration (NASA) Deep Space Network (DSN) and Korea Deep Space Antenna (KDSA) for its flight operation. In 2016, Korea Aerospace Research Institu (KARI) and NASA agreed that the KPLO would carry one NASA payload, the ShadowCam, under NASA collaboration in telecommunications and navigation for the KPLO mission. Since the KPLO utilized both DSN and KDSA, a great deal of joint effort was made between the KARI and NASA DSN teams since the beginning of the KPLO ground system design and development and continued through operational concept establishment until the real-time operation. The NASA DSN is a worldwide ground facility located in the U.S. California Goldstone, Spain Madrid, and Australia Canberra to support interplanetary missions (NASA 2023). For the KPLO mission, the Goldstone Deep-Space Communication Complex (DSCC), the Madrid DSCC, and the Canberra DSCC served as the primary service facilities during the transfer phase. Goldstone and Madrid will remain the primary service facilities, while the Canberra DSCC will be the backup for KDSA anomalies during the nominal mission phases (LEPO 2022).
The main objective of the current paper is to address the practical lessons learned from the Korea Deep-Space Ground System (KDGS), design stage to the successful operation of the KPLO, mainly focusing on the lessons learned from collaborative work with the DSN team. Therefore, the top-level DSN interface architecture for the KPLO flight operation, detailed workflows, and DSN support levels are treated in this work. Indeed, very sophisticated interface control is required to get support from the DSN (Kwan et al. 2022). Among the numerous DSN interfaces for telemetry, command, and ranging services, the current work only focuses the FD operation-related interfaces. Due to the extensive range of services provided by the DSN, listing them all here is very challenging; therefore, readers may refer to Ref. (Elliott 2020) for a comprehensive overview of the capabilities available supports from the DSN.
Through the DSN interfaces with KDGS, KPLO FD specialists, namely the navigation team, successfully conducted FD operations such as orbit determination (OD), trajectory review, maneuver planning to follow the designed reference trajectory, trajectory confirmation, and maneuver recovery.
This paper is structured as follows. Section 2 presents a summary of the results of the KDGS architecture design, including the interfaces with the DSN. This section also details the interfaces through OscarX, the Service Preparation Subsystem (SPS), and the DSN antennas allocated to support the KPLO mission. Section 3 discusses the DSN support level agreed upon between KARI and NASA DSN, providing a detailed description of the DSN’s support policy based on the support level. Section 4 describes, in as much detail as possible, the lessons learned from a practical perspective obtained for the successful operation of the KPLO. Finally, Section 5 concludes the paper. The design results and practical lessons learned in this paper can significantly reduce numerous trials and errors for future deep space missions in Korea and joint cooperative efforts with the NASA DSN.
2. KOREA DEEP-SPACE GROUND SYSTEM (KDGS) AND DEEP SPACE NETWORK (DSN) INTERFACE
To enable a highly efficient operation of the KPLO mission, KARI has designed, developed, and verified the KDGS with great success. Unlike previous ground systems that were developed and operated for low earth orbit (LEO) and geosynchronous orbit (GEO), the KDGS required much more complex operational scenarios due to its more intricate interfaces. Fig. 1 provides a simplified representation of the top-level architecture of the KDGS.
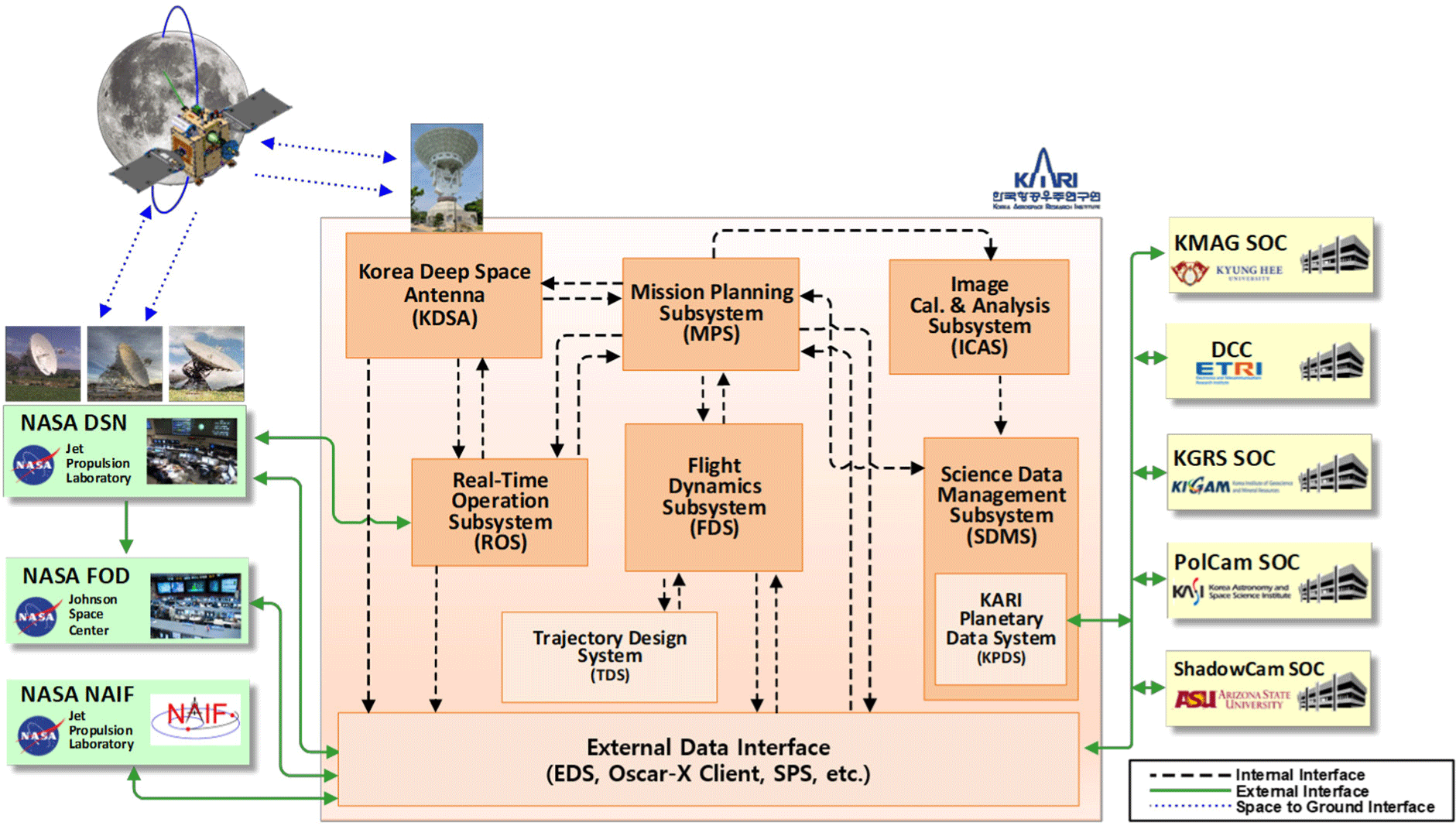
The KDGS comprises the 35-meter aperture KDSA and five major subsystems, each with unique functionalities. The Real-time Operation Subsystem (ROS), Flight Dynamics Subsystem (FDS), Mission Planning Subsystem (MPS), Image Calibration and Analysis Subsystem (ICAS), and Science Data Management Subsystem (SDMS). These subsystems function in tandem to generate various products, which are delivered to external Science Operation Centers (SOCs) through External Ground Networks (EGNs) to support the payloads. The KDGS also has interfaces with NASA, as shown in Fig. 1, with three major EGNs established between the KDGS and NASA; the Navigation and Ancillary Information Facility (NAIF), the Johnson Space Center (JSC) Flight Operations Directorate (FOD), and the DSN. These external data interfaces comprise External Data Server (EDS), OscarX client, the SPS, etc. Through NAIF, various KPLO flight data, including payload data, are parsed using the “SPICE” toolkit, which stands for S: Spacecraft, P: Planet, I: Instrument, C: Orientation, traditionally called C-matrix, and E: Event. This parsing assists scientists in planning and interpreting the KPLO data for payload operation. With NASA JSC FOD, the FD operation-related products, such as maneuver planning and OD results, are shared for Independent Verification and Validation (IV&V) between KARI and NASA. Finally, KDGS has built up external interfaces with DSN for telemetry, command, and ranging (TC&R) to support the KPLO mission operation. Among the very complex KDGS interfaces, this work will focus on the interfaces between KDGS and DSN, especially interfaces established to support KPLO FD operation. A more additional detailed KDGS design architecture can be found in Song et al. (2021).
NASA DSN has designated ten Deep Space Station (DSS) antennas, comprising primary and backup stations, to support the KPLO mission. DSS-24 and DSS-26 have been allocated for routine nominal support, while DSS-14 has been reserved for backup and contingency downlink purposes. And these DSS-24, DSS-26, and DSS-14 are all from Goldstone DSCC. From Canberra DSCC, DSS-34 and DSS-36 stations have been assigned for nominal routine support, and DSS-43 has been designated as a backup station. Finally, Madrid DSCC has assigned DSS-65, DSS-54, and DSS-56 for nominal support, while DSS-63 has been reserved as a backup station (Levesque et al. 2022). During the KPLO operation, each DSN pass was supported by one of the antennas assigned from each DSCC. In contrast, other DSN antennas not initially designated for the KPLO mission occasionally provided services, particularly when DSCC maintenance was required, or in the event of urgent requests from other missions requiring the immediate use of KPLO allocated antennas. Table 1 displays the type and size of each KPLO allocated antenna, along with its station ID and Cartesian coordinate. In Table 1, BWG refers to Beam Waveguide and HEF to High Efficiency, respectively. All Cartesian coordinates in Table 1 are based on the International Terrestrial Reference Frame (ITRF) 93 reference frame, and additional information can be found in Slobin et al. (2022).
The DSN delivers radio metric tracking data for the KPLO in either the DSN Tracking System Data Archival Format (TRK-2-34) or the DSN Tracking Data Message (TDM) format. KARI retrieves these radio metric data files from the DSN server named OscarX (O’Dea et al. 2018). The KPLO Radio Metric data files contain DSN Sequential ranging data and Total Count Phase (TCP) Doppler data. Access to the OscarX server is restricted to authorized users, and authorization can be obtained through the Mission Interface Manager (MIM). MIM is a DSN manager responsible for the DSN interface to specific customers (O’Dea et al. 2018). During the preparation for the KPLO operation, DSN agreed with KARI to provide both the TRK-2-34 and TDM data through OscarX. Initially, KPLO attempted to use only the TDM format for its FD operations. However, there were internal concerns that the Consultative Committee for Space Data Systems (CCSDS) standard TDM format might be unstable in processing DSN data with FDS. Accordingly, it was decided to receive both TDM data and the TRK-2-34 format, which has been traditionally and stably used thus far (Kwan et al. 2022). While operating the KPLO, the stability of the DSN TDM data was confirmed and showed no differences in OD performance when compared to the DSN TRK-2-34 data format.
DSN delivers the TRK-2-34 and TDM files in two different forms specified by the delivery point. One form is the “quick look” file, also known as the partial file. Partial files are delivered at customer-specified interval times, typically set to 60-minute intervals per tracking pass. The other form is the “complete file.” Complete file products are delivered at the end of each DSN pass after the final station calibration. After major events such as the KPLO’s separation from the launch vehicle or other numerous maneuver execution events, the partial files played significant roles in near-real-time OD. Dependent on the importance of the mission phases, the delivery interval of the partial files can be adjusted upon the customer’s request as long as the requested intervals are within the limits of DSN support.
The high-level DSN tracking data flow is shown in Fig. 2, which portrays the path that the tracking data takes from the DSCC to the JPL Deep Space Operation Center (DSOC) and eventually to the KARI navigation team. The tracking data is first acquired by the DSCC and then delivered to the DSOC for storage. Subsequently, the KARI navigation team securely retrieves the stored tracking data from OscarX using the secure File Transfer Protocol (sFTP) (Levesque et al. 2022). The abbreviations presented in Fig. 2 denote the various stages of the DSN tracking process, including Downlink Tracking & Telemetry (DTT), Data Capture and Delivery (DCD), Tracking (TRK), and DSN Uplink Subsystem (UPL).
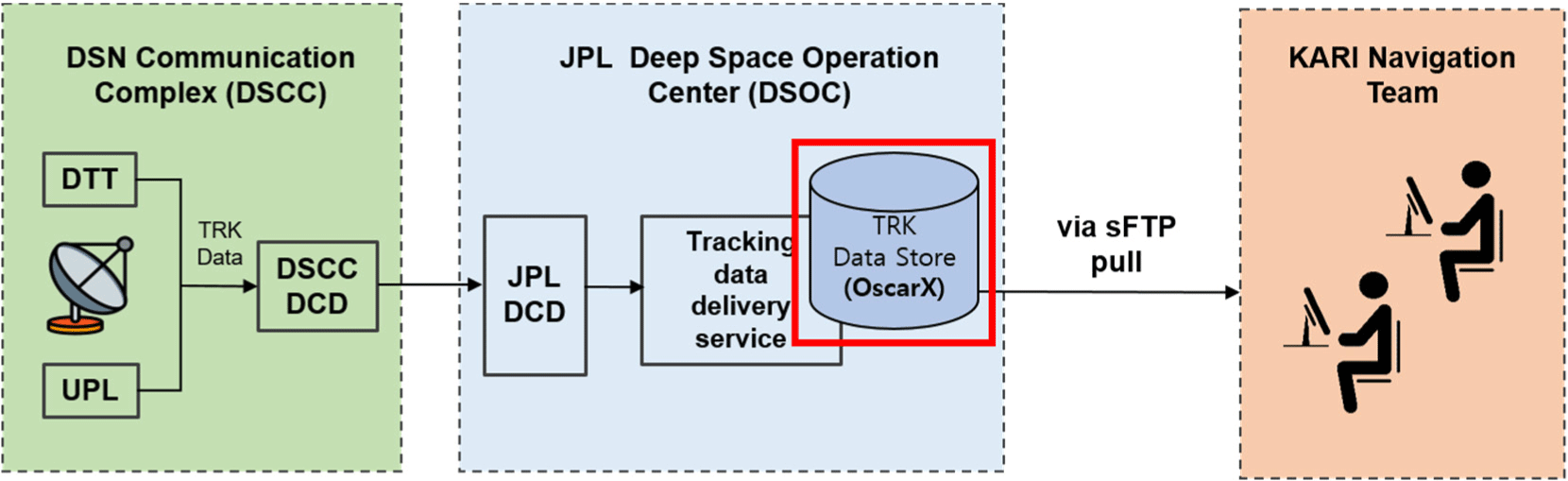
In the previous section, OscarX was described as a DSN tracking data server. However, in order for a customer to receive support from DSN, a suitable interface is required. The system responsible for providing this interface is called the SPS. The SPS serves as a software interface for mission customers to plan and prepare for DSN tracking support. It includes a web portal and data repository for support products, as well as an interface for scheduling DSN resources. The SPS generates a Support Data Package (SDP) for each scheduled track, utilizing input files for the mission and DSN configuration tables (Elliott 2020).
The SDP consists of files containing antenna pointing, telemetry predictions, and a Pass Sequence of Events (PSOE) presenting a timeline of events during the pass. These files are essential for automating pass operations by the DSN, eliminating the need for manual intervention by the station link controller. The SPS portal hosts all these products, which are available online for up to six months of historical data. The SPS portal offers several products relevant to the mission interface, including DSN tracking schedules, which can be accessed through custom files via SPS queries or 7-day schedule files. The portal also provides View Period (VP) files, which offer information on spacecraft visibility with DSN stations, SDP files containing PSOE files for automating station operations, DSN Mission-specific station configuration files, and Mission ephemeris and Mission Nominal Sequence of Events (NSOE)/DSN Keyword Files (DKF) files (Elliott 2020). It is important to note that this paper only deals with the interface with the SPS from the perspective of the navigation team, among the many functions of SPS. Readers can refer to Elliott (2020) for more details.
Customers can upload predictive ephemeris in two formats to the SPS to schedule DSN tracking: Spice Kernel (SPK) format and Orbital Ephemeris Message (OEM). In addition to using one of these formats, customers must upload the following two predicted ephemeris files to the SPS portal: scheduling grade and predicts-grade ephemerides. The scheduling grade ephemeris is mainly used to generate a VP file for DSN scheduling and must be maintained on the portal for at least 548 days (18 months) at all times. They should be delivered at least every six months or more frequently if accurate scheduling is required. Meanwhile, the predicts grade ephemeris is used in the SDP generation, which is delivered to the station to provide information about the spacecraft trajectory during the pass. The predicted grade ephemeris should be at least three days in duration, but there is no specified maximum duration. Additionally, ephemeris files can be submitted at any time, and it is not necessary to deliver a separate file for each track. It is important to note that the upload frequency, prediction duration, and other specifics may change to maximize the operations’ efficiency.
The frequency of uploading predictive ephemeris with different prediction spans also depends heavily on the different mission phases. In the case of KPLO, the navigation team closely collaborated with the DSN team to provide optimal input for each mission phase. For instance, during KPLO’s initial acquisition, the KARI team provided predicted ephemeris files for the first five daily launch opportunities delivered by launch–10 days along with predicted ephemeris for launch anomaly. In the event of a launch recycle, the KARI navigation team maintained an additional five days of opening launch opportunities in the SPS. DSN generated and delivered pre-launch SDP to the supporting station by launch–2 days. When a off-nominal launch occurred, the KARI navigation team uploaded updated predictive ephemeris to the SPS as soon as possible. Once new files were received, it took approximately 35 minutes for DSN to generate, transmit, and load the new SDPs (Elliott 2020).
For the nominal mission phases, the KPLO navigation team uploads scheduling and predictive grade ephemerides to the SPS. The scheduling grade ephemerides cover a span of approximately seven months and are updated on the first Wednesday of each month or after maneuvers. These ephemerides always include any maneuvers that occur within the span. Therefore, the predictive grade ephemerides and a Long Term Maneuver Plan (LTMP) file generated by the KARI navigation team are provided to the DSN together for the scheduling grade ephemerides. The predictive grade ephemerides cover a period of at least 7 days by default (currently delivers 3 weeks as in lunar mission orbit), and if a maneuver is scheduled during this period, the relevant information is also delivered to the DSN. Detailed information about the upcoming first maneuvers, including the start and stop times and direction of the maneuver, is provided to the DSN via the Maneuver Request (MNRQ) file generated by the KPLO navigation team. The LTMP and MNRQ files are entirely different file formats. MNRQ files are more detailed and complicated versions to show the maneuver information compared to the LTMP, which contains detailed characteristics of a single upcoming first maneuver. However, LTMP file is a more simplified version of MNRQ that contains more future maneuver information. It’s important to note that the delivery frequency and duration of these predictive ephemerides can vary during operations, so it’s crucial to establish a system that can effectively adapt to these changes.
3. DEEP SPACE NETWORK (DSN) SUPPORT LEVEL FOR THE KOREA PATHFINDER LUNAR ORBITER (KPLO) MAJOR EVENTS
The DSN offers tracking support at varying intensity levels, with four “support levels” available. The default or nominal level of support is referred to as L4. However, in circumstances where the utmost reliability is required, such as a launch, landing, or orbit insertion, where a malfunction in the ground data system could endanger the spacecraft and potentially terminate the mission, L1 support is employed. For events at L1 or L2 levels, there is a formal risk assessment review of the mission risks, and DSN support plans in collaboration with the mission and a DSN Review Board. Missions must notify the MIM one year in advance of any mission-critical event that may require elevated support at L1 or L2 levels. The decision to provide L1 or L2 support will necessitate the DSN Project Office’s concurrence. On the other hand, L3 elevated support will be decided jointly by the MIM and the Network Operations Project Engineer (NOPE) with the approval of Office 927 Service Management and Operations (Elliott 2020). In Table 2, the required DSN support activities are listed for each different DSN support level. For events designated as “(X),” the requirement is at the discretion of the MIM and NOPE (Elliott 2020).
After several discussions with the DSN team, KPLO has decided to receive the following tracking requirement and DSN support levels for each different mission phase, as shown in Table 3 (Levesque et al. 2022). The abbreviations shown in Table 3 are as follows: Trajectory Correction Maneuver (TCM), Lunar Orbit Insertion (LOI), Orbit Trim Maneuver (OTM), and Orbit Maintenance Maneuver (OMM), respectively.
Table 3 indicates that the DSN support level for most of the KPLO events is either L3 or L4, except for initial acquisition and checkout, TCM#1, and LOI#1 events. Specifically, the DSN support level for the initial acquisition and checkout and TCM#1 events were L1 and L2, respectively, and for the LOI#1 event, it was L2. To comply with the DSN support level requirements, KARI and DSN team conducted a formal risk assessment review, and a DSN Mission Event Readiness Review (MERR) was held at least 30 days before the initial acquisition and checkout as well as TCM#1 events (Levesque et al. 2022).
Similarly, a DSN-Mission Event Level 2 Readiness Review (L2RR) was conducted at least 30 days before the KPLO LOI#1 event to evaluate the mission risks and DSN support plans (Johnston et al. 2023). For L1 and L2 support, the NOPE will issue a Briefing Message at least five days before the event, while L3 support may involve an informal DSN-Mission Status Review at the MIM and NOPE’s discretion. In the case of L3 support, a Briefing Message will be sent three days before the event. For further details on DSN support levels and procedures, readers can refer to Elliott (2020).
4. PRACTICAL LESSONS LEARNED
Through the KPLO mission, KARI collaborated with the NASA DSN team for the first time. While the KARI had much experience in operating spacecraft in LEO and GEO, this collaboration allowed KARI to learn and acquire many aspects of spacecraft operations for lunar exploration and future deep space missions. The operation of deep space exploration missions required more systematic risk management than the KPLO operation team had initially anticipated, and this required a significant amount of well-trained personnel resources. In particular, the joint review with the DSN team, namely MERR, and L2RR, was conducted in a very systematic manner, and the KPLO operation team required more preparation time than anticipated. During the joint review meetings, all aspects of the preparation for the successful operation of KPLO were covered. This included the status of implementation tasks, facilities, operational systems, procedures, and operations personnel support. Specifically, the joint review meetings included the results of DSN verification and validation training and testing, interface testing between the KPLO Mission Operation Center (KMOC) and DSN, RF compatibility testing between KPLO and DSN, mission service training activities, ground data system testing, KPLO Delay Tolerant Networking (DTN) testing, and Operational Readiness Tests (ORTs) such as voice rehearsals with DSN participation. A significant amount of documentation was prepared jointly, and the status of document preparation was also discussed. Additionally, contingency plans for critical events during actual operations were prepared and discussed during the review meetings. The overall collaboration work required a lot of discussions and mutual understanding than the KPLO operation team had firstly anticipated.
From the perspective of the KARI navigation team, additional system review meetings with the DSN team were an extra burden on top of the already ongoing activities of developing, verifying, and preparing ground systems for FD operations suffering from a shortage of human resources. This was because the KARI navigation team had to produce and share several analysis results correctly within a limited timeframe just before the KPLO launch, which was unexpected and had not been accounted for. Despite such circumstances, thanks to the DSN team’s dedicated collaboration, the operations of KPLO are being successfully carried out. Through the KPLO mission, KARI has acquired and assimilated many essential elements requisite for collaboration with DSN. Korea is currently planning additional deep space exploration missions for the future. Based on this experience, it is anticipated that KARI and DSN will be able to collaborate more seamlessly in the future and that the practical lessons learned from the collaboration on KPLO will mitigate errors and inefficiencies in future endeavors. Thus, it is very important to adapt these experience when preparing the next space mission.
The quality of the DSN tracking data exceeded the expectations of the KARI navigation team. The KARI navigation team conducted numerous simulations and rehearsals until just before the launch of KPLO to ensure successful operation. During simulations and rehearsals, DSN tracking measurements were simulated and set to have characteristics, such as biases and white noise, as similar as possible to the actual DSN data (Song et al. 2022). However, when KPLO was launched, and DSN tracking measurements were received for OD, surprisingly, the precision of DSN tracking measurements exceeded the way over to the KARI navigation team’s first expectations. Despite the excellent quality of DSN tracking measurements, it is important to check certain aspects when performing OD using DSN tracking measurements. As mentioned earlier, DSN provides tracking data in both a quick-look file, a partial file, and a complete file, which is the final version of tracking measurement after station calibration. The partial file is immediately provided after tracking and helps in performing near real-time OD. However, there are occasional cases where the file size of the final version tracking measurements received by OscarX may differ from that of the partial file. Therefore, the size and quality of tracking measurements should be checked after retrieving from OscarX and reported, if necessary, to DSN as soon as possible. If there is an error in the tracking file itself, the DSN team will perform station calibration again and provide an updated file to support the OD performance enhancement. Actually, the uncertainty level of range measurements from DSN is found to be around 0.5 m (1-sigma). Furthermore, careful monitoring of the provided tracking measurement quality is necessary when there is a change in the DSN tracking complex, such as from Goldstone DSCC to Madrid DSCC. Nevertheless, there was no need to worry too much because as the operation continued, the quality of the tracking measurements was gradually stablized. However, when there is a DSN station check, replacement of various equipment, or other tests conducted in DSN facilities, it is necessary to carefully examine the quality of the tracking data and cooperate closely with the DSN team.
As mentioned in Section 2.4, SPS is essential to obtain DSN support. The KARI navigation team has prepared predictive ephemeris data from KPLO in both .spk and .oem formats for upload to SPS to ensure smooth DSN support. However, we experienced various trials and errors while preparing for actual operations. Initially, the KARI navigation team attempted to generate .spk files and directly upload them to SPS. However, errors occurred every time when tried to upload the files. After consulting with the DSN team, it was confirmed that the file format accepted by SPS is not strictly .spk but the Spice Transfer (*.xsp) format. In other words, uploading .xsp files to SPS will automatically convert them into .spk files instead of directly uploading .spk files to SPS. The KARI navigation team attempted to develop software to convert .spk files back to .xsp files. However, since SPS also accepts .oem files for upload, it was decided to upload .oem files directly instead of .spk files. However, uploading .oem files to SPS was not entirely smooth either. When uploading .oem files to SPS, the system often encountered “space ratio errors.” When creating .oem files, the file size is often large, so variable step sizes are used to generate the file. In other words, .oem files are automatically divided into several segments depending on the integration accuracy of the predictive ephemeris. This “space ratio” error is a type of issue that arises in highly dynamic regions of orbit or trajectory. For example, the segments before and after a maneuver are composed of relatively dense step sizes in the .oem file. However, the segments where the rate of change of the predictive ephemeris is not significant, .oem files are composed of larger integration steps. The “space ratio” error occurring in SPS was indeed due to the step size issue. When the KARI navigation team uploaded .oem files to SPS, the SPS automatically converted the file to .spk format and returned “space ratio” errors during the conversion. The “space ratio” error is a type of constraint given when converting .oem files to .spk files and is intended to prevent a decrease in precision due to interpolation algorithms during the generation of predictive ephemeris. This error can be resolved by either splitting one file into multiple files and uploading them to SPS or by creating the file in advance to avoid “space ratio” issues. There were many internal discussions on how to resolve this issue. Finally, the KARI navigation team chose not to split the file into multiple pieces for uploading, because the KPLO navigation team could not know in advance how many files should be needed to upload the predictive ephemeris with splitting. Instead of splitting one file into multiple pieces, additional software was urgently developed that could detect the possibility of a space ratio error before uploading the .oem file to SPS. This software allowed for smooth .oem file uploads during actual FD operations.
One aspect that the KARI navigation team should have paid more attention to during the first collaborative work with the DSN team was the frequent changes in DSN schedules. Frequent DSN schedule change is totally understandable since the DSN actually supports a significant number of space exploration missions under minimal DSN resources. The changes in DSN tracking schedules arise from a wide range of causes, such as unexpected mission failovers, station maintenance due to problems with the DSN station itself, and frequent requests for schedule adjustments when new missions are launched. During the operation of KPLO, there was a tracking schedule adjustment request due to issues with the Cislunar Autonomous Positioning System Technology Operations and Navigation Experiment (CAPSTONE) mission failover, setting the launch schedule for the Artemis 1 mission, etc. Whenever such requests were received, the KARI navigation team analyzed the feasibility of accommodating the request and responded to DSN. If the requested schedule changes were not critical for KPLO’s mission performance, most of the request was accepted. However, if there was a KPLO critical event or if there was a significant impact on KPLO’s OD performance due to the schedule change request, the KARI navigation team carefully analyzed the impact and worked together with the DSN team to minimize the overall impact, not only for the KPLO but also to other missions as much as possible.
In Fig. 3, an example of KPLO’s working schedule is shown, which is periodically updated and provided by DSN. The working schedule shown in Fig. 3 includes all information on which antenna will be used for KPLO’s tracking, and the sheet is updated every time when the relevant schedule is updated from DSN. Therefore, this schedule sheet must be constantly updated to the latest version for operators to refer to during actual operations. The working schedule shown in Fig. 3 only deals with KPLO; there may be a need to identify the additional availability of DSN antennas in case of requesting additional tracking schedules from DSN. The DSN schedule visualization provided by SPS Web (CalTech 2023), shown in Fig. 4, can be used to quickly view the schedules for current and upcoming DSN antennas’ support for the planned other missions. By utilizing the schedule visualization provided through the SPS web, operators can not only keep track of KPLO’s tracking schedule but also gain an overall understanding of the DSN’s schedule status, which allows more seamless collaboration with DSN. Indeed, the process of responding to, analyzing, and collaborating with the DSN team on schedule changes was an additional workload for the KARI navigation team. Indeed, this additional work was not anticipated at all before the launch of the KPLO. It required a significant investment of time and effort. If KARI cooperates with the DSN in the future, the aspect of tracking scheduling management should always be addressed.
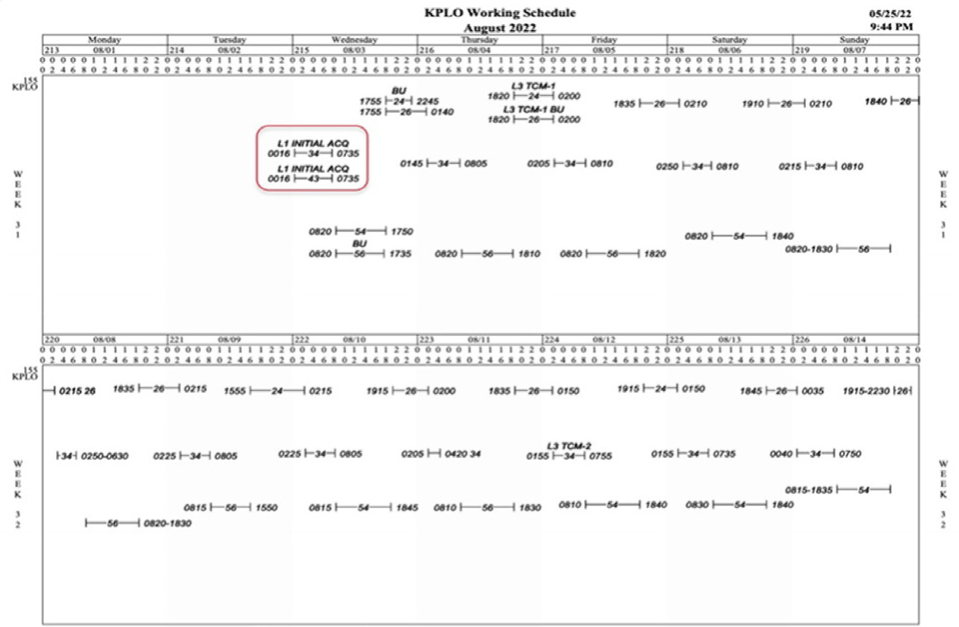
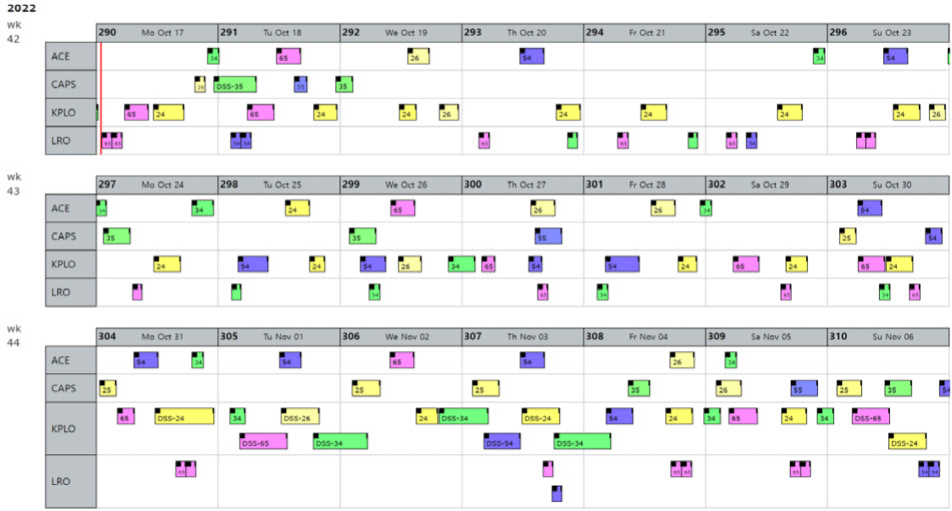
5. CONCLUSIONS
Lessons learned through the DSN collaborative work made during the KPLO mission journey, starting from the early design of KDGS until the full operation, are addressed in this work. Starting from the top-level interface architecture between KDGS and DSN, and the primary systematic interface required to be supported by DSN as a customer, such as OscarX and SPS, are summarized. In addition, the critical aspects of the DSN support level while collaborating with the DSN team are also treated. Most importantly, practical lessons learned from actual flight operations experience are summarized. According to the experience of the KPLO navigation team, collaboration with the DSN team involved a significant amount of additional work that was not initially anticipated. To undertake future deep space exploration missions with DSN, including Korea, it is essential to secure human resources prepared for the relevant tasks as there will be another level of professional work awaiting.
Smooth use of the OscarX and SPS interfaces is crucial for operational stability when missions are supported by DSN. It is essential for operators to have a sufficient understanding of the characteristics of both systems and to prepare for operation through interface testing. This is not only essential for efficient flight operations but also crucial for working as a joint team with the DSN team. During operation, it is recommended to check the size and qualitative content of tracking data received from OscarX, especially during the critical phase of the mission. When uploading predictive ephemeris to the SPS, it is recommended to always check that uploaded files are free of errors, even containing states in highly dynamic regions. Providing sufficient extra information to the DSN ahead of the major critical mission event, such as detailed maneuver information, is essential to secure reliable support from the DSN. Given the nature of DSNs’ need to support multiple missions with limited resources, it is essential to have the ability and resources to respond proactively to frequent requests for tracking schedule changes. The SPS Web provided by DSN can be useful for understanding DSN resources regarding such schedule changes, and experienced operators adapted to this environment are also essential for collaborating with the DSN team.
The KPLO mission marked the first collaboration with the DSN in Korea’s space history. The KPLO operation team faced numerous challenges and overcame many trials and errors in successfully operating KPLO with the DSN team. Through this experience, the overall KPLO operation team was able to gain crucial insights and develop important considerations for future deep space exploration missions. These practical lessons can serve as a valuable resource for future collaborations with the DSN. The partnership between KPLO and DSN teams has also contributed to the establishment of mutual understanding and trust, providing a solid foundation for future cooperation.