1. INTRODUCTION
The Korea Pathfinder Lunar Orbiter (KPLO) is Korea’s first lunar exploration mission (Ju et al. 2013). The KPLO will be launched in the middle of 2022 and delivered at 100 km altitude lunar polar orbit. The details and recent progress of the KPLO mission were found in several pieces of literature (Ju et al. 2013;Choi et al. 2018;Davis et al. 2018;Song et al. 2018;Bae et al. 2020;Hong et al. 2020;Kim et al. 2020;Lee et al. 2020;Park et al. 2020;Song et al. 2020). For the successful mission operation and payload data acquisition of KPLO, the ground system has an important role. The Korea Deep Space Ground System (KDGS) is developing to support KPLO system rehearsal and mission operation. Korea Deep Space Antenna (KDSA) is a 35 m dish antenna for tracking and communicating with space exploration spacecraft such as KPLO. Real-time Operation Subsystem (ROS) generates and sends the telecommand to an antenna and receives telemetry for monitoring the status of KPLO. Payload Storage Server (PSS) generates the product files of payload observation and examines receiving status. Flight Dynamics Subsystem (FDS) performs state prediction, orbit determination (OD), maneuver planning, fuel accounting, and orbital events prediction. The KPLO mission timeline and mission commands are generated by the Mission Planning Subsystem (MPS). MPS also provides payload mission management and image acquisition planning such as LUTI and ShadowCam and contact schedule generation. Image Calibration and Analysis Subsystem (ICAS) performs LUTI data calibration and level product generation. Science Data Management Subsystem (SDMS) delivers the science data to Korean science instrument developers, releases the science data products to the public, and stores. Disturbance Tolerance Network Node (DTN Node) interfaces with DTN Control Center and ROS to support DTNPL operation. External Data Server (EDS) exchanges mission planning products between KDGS and Science Operations Center (SOC) such as Korean payload SOCs and NASA ShadowCam SOC. For visual information of mission operation, the KPLO operation visualization function is also provided.
For the flight dynamics operation, tracking support conditions of ground stations for OD is critical in determining the spacecraft’s navigation performance. The maximum tracking support delivers the best navigation solution; therefore, full support of possible ground stations is essential during the critical event of the mission, such as the launch phase and orbit maneuver. However, tracking resources such as station availability, elevation cut-off angle constraints, and tracking measurement accuracy can be limited for the lunar missions. A tracking gap can be occasionally occurred due to the failure of the station. Therefore, a backup plan for tracking missing periods may be needed. For the stable ground tracking support and optimal resource distribution, the tracking requirement should be finalized considering various situations. In previous lunar missions such as Lunar Reconnaissance Orbiter (LRO), Lunar Atmospheric Dust Environment Explorer (LADEE), Gravity Recovery and Interior Laboratory (GRAIL), and Beresheet, various factors such as mission phases, OD requirements, and tracking constraints were considered for selecting tracking support levels of spacecraft (Nicholson et al. 2010;Ryne et al. 2013;Policastri et al. 2015;Shyldkrot et al. 2019). For example, the NASA’s GRAIL mission, which has two orbiters around the Moon, was supported by six primary and seven secondary antennas of three deep space network (DSN) complexes. The tracking request for the science and extended mission phases was one pass per day per each orbiter with no gaps longer than sixteen hours (Ryne et al. 2013). For the trans-lunar phase of the GRAIL mission, one pass per two days, which has an eight-hour duration, was supported (You et al. 2012). Therefore, the navigation performance analysis for various tracking support conditions by ground stations should be prepared for the preliminary analysis of the KPLO mission.
Numerous studies of KPLO OD has been progressed and delivered useful results for both Earth-Moon translunar trajectory and lunar mission orbits. Arc-length selection strategies for both phases were investigated for better orbit accuracy (Kim et al. 2018a;Kim & Song 2019). The OD simulations for the total KPLO mission duration were established (Kim et al. 2019). The OD analysis for weak stability boundary/ballistic lunar transfer trajectory was also presented for the changed trajectory of the KPLO mission (Kim et al. 2020). For the lunar mission orbit, KPLO OD performance can be mainly affected by ground supporting conditions. The effect of the selection of lunar gravity models and supporting the number of stations was investigated for the KPLO OD in mission orbit (Kim et al. 2018b). Most of the KPLO OD studies in the lunar mission orbit assumed ideal tracking support, which tracks every lunar orbit pass by three stations. Therefore, actual tracking conditions and the performance variations by ground support condition should be analyzed to prepare KPLO mission planning and operation in the lunar mission orbit. In this study, the results of the KPLO OD analysis considering ground tracking support conditions were accomplished. Various conditions such as daily tracking frequency, elevation cutoff angle, tracking measurement accuracy, and tracking gap caused by station failure are considered, and their OD and orbit prediction (OP) performance variations according to support conditions were investigated.
Section 2 described the method of measurement simulation for OD analysis considering ground tracking support conditions. The OD methods and strategies such as modeling setting, arc length configuration, and orbit quality assessments are summarized in Section 3. Section 4 includes the OD analysis results considering four ground tracking support conditions. Section 5 presents the conclusion of this research.
2. MEASUREMENT SIMULATION
For the measurement simulation of the KPLO in mission phase, the lunar polar orbit with a 100 km altitude was generated as a reference orbit using initial orbit information, as shown in Table 1. The epoch time is January 1, 2023, at 00:00:00 (UTC), and orbit is propagated for one month (2023/1/1–1/31). Apoapsis altitude and periapsis altitude, inclination, right ascension of ascending node (RAAN), an argument of periapsis, and true anomaly values are represented in Moon true of date (TOD) coordinate frame as shown in Table 1. The dynamic modeling setting for the generation of reference orbit is summarized in Table 2. For the lunar gravity modeling, the GL0900D model was used with a degree of 200 and an order of 200 (Konopliv et al. 2014). The JPL DE430 is applied for the planetary ephemeris information. Solar radiation pressure is applied, and the third body effect by Earth and Sun is considered. For numerical integration, Runge-Kutta 7–8 with a variable step is used.
The range and Doppler measurements were generated at 60 seconds intervals using simulated reference orbit and three ground stations, consisting of 2 DSN antennas (Goldstone and Madrid) and KDSA (Yeoju). The measurement errors were added using the KDSA specification and the DSN service catalog (JPL 2015). We used the same statistical values as Kim et al. (2019). For normal cases, measurement noise values of the DSN range and Doppler are set to 13 m and 0.003 Hz, respectively. It is assumed that the KDSA has noise values of 22 m and 0.15 Hz for range and Doppler measurements, respectively (Kim et al. 2018a).
In this study, various ground tracking configurations were considered for the measurement simulation because of case studies for the four tracking support conditions. For the analysis of the daily tracking frequency effect on KPLO OD, three tracking-arc configurations are applied. Fig. 1 and Table 3 show the configuration of the measurement tracking frequency effect investigation. In case of the KPLO mission orbit, each pass has about 2-hour duration for antenna pointing because 100 km orbiting altitude lunar orbit has about 2-hour orbit. We prepared three configurations such as case I (full coverage), case II (67% coverage), and case III (33% coverage) as shown in Fig. 2 and Table 3. For the case I (full coverage) condition, each DSN antenna has four passes per day, and KDSA has four passes per day tracking time. Case II (67% coverage) has two passes per day of each DSN antenna and the same KDSA passes as case I, and case III (33% coverage) has two passes per day condition for both DSN and KDSA. The actual tracking duration of each pass is limited to the 30-min duration for each lunar orbit among total 2-hour duration. For the investigation of tracking accuracy effect on KPLO OD, three cases such as normal condition (case I), 30% degradation (case II), and 50% degradation (case III) of tracking accuracy were configurated in this study. For tracking accuracy degradation cases, 30% and 50% larger noises than normal case were added. For the analysis of the tracking failure effect on KPLO OD, three cases such as no missing (case I), KDSA 1 day missing (case II), and KDSA 2 day missing (case III) were prepaed, as shown in Fig. 3 and Table 4. For the configuration of tracking missing condition, we simply excluded the tracking of KDSA.
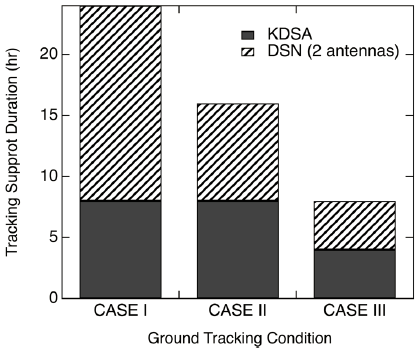
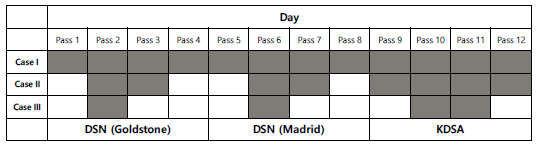
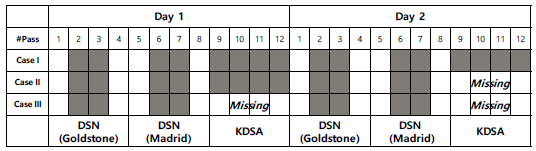
3. ORBIT DETERMINATION
In this section, the OD settings and estimation strategies were summarized. For OD of KPLO mission orbit, a sequential estimation method using an extended-Kalman filter and backward smoother is utilized. Daily OD using 48-hours tracking arcs was performed. After OD, OP for 48-hours was additionally followed. The dynamic modeling configuration for OD in lunar orbit is summarized in Table 2. Most of the dynamic settings for reference orbit generation were maintained except the lunar gravity model and degrees and orders. For OD and OP analysis, we used the GL0660B gravity model with a degree of 100 and an order of 100 (Konopliv et al. 2013). For measurement modeling, tropospheric reflection and ionosphere delay were included, and the plate motion of tracking station and antenna correction were considered. Measurement and time bias were estimated in the OD procedure. Estimation parameters consist of position and velocity of KPLO, solar radiation pressure coefficients Cr, and transponder bias. The iterative fine-tuning of the OD filter was not performed in this study. The station-keeping maneuvers for maintaining an altitude of KPLO and wheel-off loading maneuvers for the momentum desaturation were not considered in this analysis. For the orbit quality assessments, orbit uncertainty by error covariance, orbit overlaps differences, and orbit differences between true and estimated orbits were used. The root mean square (RMS) values of 3D position were checked, and OD and OP qualities for the various ground support conditions were finally evaluated by the mean and standard deviation values. In this study, OD for various ground tracking support conditions such as daily tracking frequency, elevation cut-off angle, tracking accuracy, and tracking failure was carried out.
4. RESULTS
In this section, OD and OP for KPLO mission orbit were performed to investigate various ground supporting effects such as daily tracking frequency of station, elevation cutoff angle, tracking measurement accuracy degradation, and tracking failure of the station. Detail configuration for investigation of each effect is described, and OD and OP results and performance analyses are demonstrated. For the KPLO mission, 160 m (3D position RMS, 3 sigma) is the OD requirement in the mission phase. For the OP requirement, the value of 1.7 km (3D position RMS, 3 sigma) for 48 hours is given for the support overall KPLO mission operation. These are a critical guideline to evaluate the ground supporting effects.
In lunar orbit at an altitude of 100 km, one orbit cycle duration is two hours. It means that twelve passes per day exist. In previous analyses, KPLO OD for the lunar mission phase was attempted using ideal tracking support (tracking for every orbit), which has eight passes per day for two DSN antennas and four passes per day for KDSA (Kim et al. 2018; Kim et al. 2019). In the real situation of the mission, each station's daily tracking frequency can be adjusted by ground supporting resource conditions. In this subsection, three daily tracking frequency cases are assumed for the consideration of practical support conditions.
-
• CASE I: DSN (8 passes per day), KDSA (4 passes per day)
-
• CASE II: DSN (4 passes per day), KDSA (4 passes per day)
-
• CASE III: DSN (2 passes per day), KDSA (2 passes per day)
CASE I is a full tracking support condition (tracking for every twelve passes). Each station has four tracking passes per day for KPLO’s orbit. For CASE II, the DSN’s tracking support is shortened to half coverage from eight passes to four passes, and tracking support of KDSA is the same as CASE I. In CASE III, both DSN and KDSA tracking support reduced from four passes to two passes. In fact, each antenna of DSN has only one pass per day in CASE III. Details of tracking support condition of the KPLO in lunar orbits according to daily tracking frequency are shown in Fig. 2. We can check that all twelve passes are divided into three parts for two antennas of DSN and KDSA. In Table 3, we also see the total support hours and percentage of ground support. For tracking one orbit of KPLO, each antenna should be assigned two hours whether the orbit is tracked or not observed.
Fig. 4 and Table 5 show OD and OP performance results according to tracking support condition, daily tracking frequency. The OD and OP results are represented the RMS values of the 3D position. It is clearly found that the OD and OP performance become worst under poor tracking support circumstance, which is CASE III (total four passes per day by three stations). Especially, substantial error peaks of OD and OP errors, which are not satisfied with the OD requirement of the KPLO mission, are observed in some daily OD arcs (1/13 OD and 1/29 OP), as shown in Fig. 4. The 3D RMS values of 1/13 OD position differences between estimated and true orbits reach 254 m, which break the 160 m position requirement of KPLO OD. The overlap precision for January 12, which is the differences between the overlapped period of 1/13 and 1/14 OD solutions, has the 3D RMS values of 294 m. For overlaps precision of January 28 and 29, 3D RMS values have 170 m and 230 m, respectively. For 48 hr prediction accuracy, we also found that less frequent tracking under a-third ground support can deliver significant large degradation. Fortunately, the results of OP are maintained under the OP requirement of the KPLO mission. However, it has the possibility to break the requirement of OP on another date.
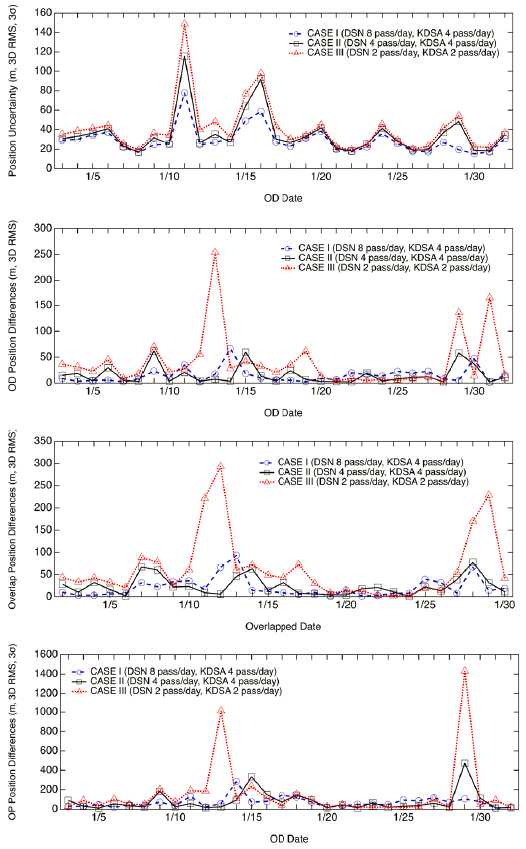
Meanwhile, we can find that the performance difference between CASE I and II is not significant. Table 5 summarizes the position accuracy statistics for different daily tracking frequency conditions. For the three tracking support conditions, daily OD arcs' mean and standard deviation values during one month were investigated. For CASE I, which is a full supporting condition, mean and standard deviation values have the best performance among the three conditions. The OD accuracy differences between CASE I and II are not significant, and the OP performance of CASE II has a large standard deviation value due to 1/29 OD, as shown in Fig. 4. All position accuracy statistics of CASE III deliver the worst performance. As a result, we concluded that tracking supports more than eight passes per day was recommended for the stable operation of KPLO in lunar orbit. For the investigation of elevation cut-off angle effect, tracking accuracy degradation, and tracking failure effect, CASE II, which consists of four passes per day for both DSN and KDSA, is used as a normal tracking condition.
The elevation cut-off means the masking for the low elevation angle of the tracking pass. Tracking capability can be limited by hardware constraints or station environment restriction. If the ground station tracks spacecraft at a lower cut-off elevation angle, the amount of tracking data increases. However, a low elevation angle means more passing time of atmosphere, and therefore they may include more errors caused by atmospheric refraction. The selection of a cutting angle for the tracking data is one of the strategies for the improvement of navigation performance. For the investigation of the elevation cut-off angle effect on OD, two cases, which are five degrees and ten degrees, were applied in this subsection.
Fig. 5 shows the differences between OD and OP results of five and ten degrees of elevation cut-off angle. Overall, the performance difference caused the selection of elevation cut-off angle is not significant. Position uncertainty and overlaps precision did not affected by elevation cur-off angle values for tracking pass. It is observed that OD and OP position differences improved by higher elevation cutting angle at a few arcs (OD dates of 1/6, 1/9, and 1/15). For the OD date of January 30, OD and OP performance of lower elevation cut-off angle, five degrees, delivers better results. We can check that the higher elevation cutting angle does not always deliver better accuracy. Table 6 shows the position accuracy statistics for different elevation cut-off angles. We confirmed that position accuracy variations according to the elevation cut-off angle are not significant. As a result, we can conclude that the effect of the elevation cut-off angle for OD and OP performance is not critical. In some cases, ten degrees of elevation cut-off angle can deliver better accuracy, and therefore it is more profitable to use a higher elevation cut-off angle for processing tracking data. For the measurement accuracy effect and station missing effect, we applied ten degrees for the elevation cut-off angle.
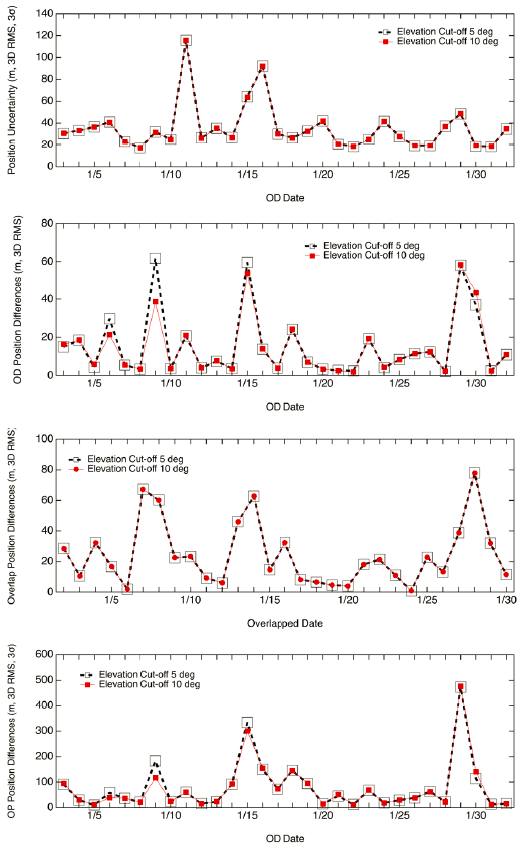
The measurement accuracy of the tracking station typically has guaranteed values. However, tracking accuracy can be degraded due to hardware error, tracking geometry, transponder characteristics of spacecraft, weather conditions, ionospheric delay, troposphere refraction, and solar activity. In this subsection, we assumed that tracking measurements of KPLO were degraded 30 and 50 percent compared to normal tracking conditions. To investigate the tracking accuracy degradation effect, we additionally simulated two cases of measurements by adding 30 and 50 percent larger errors for range and Doppler tracking data as follows:
-
• CASE I: Normal tracking accuracy
-
• CASE II: 30% accuracy degradation
-
• CASE III: 50% accuracy degradation
Fig. 6 and Table 7 show the OD and OP performance results according to tracking measurement accuracy degradation level. For the case of position uncertainty, the performance of all cases is placed at similar levels. At the end of January, a 50 percent degradation case delivers the worst uncertainty, but it is not meaningful. For the OD position differences between estimated and true orbit, 50 percent degradation case (CASE III) delivers the worst accuracy, as shown in Fig. 6. The OD position differences and overlap precisions of CASE II present a similar performance with CASE I except one arc (1/31). For 48 hr prediction performance, a similar trend with OD position differences is observed, but unusual cases are also found at 1/29 OD date. The OP accuracy is affected by state accuracy at the epoch of prediction. Therefore, it has the possibility to have large errors around the epoch of the prediction period in the case. Table 7 summarizes the position accuracy statistics for different tracking accuracy degradation cases. The mean values of CASE I and II have similar levels, and the standard deviation values of 30 percent degraded case have larger dispersion than a normal case. The 50 percent degraded measurement case (CASE III) has the worst OD and OP performance. However, these effects are not dominant to lead OD and OP accuracy of KPLO among various considering parameters because several arcs yield a large dispersion. As a result, we concluded that CASE III delivers unstable OD and OP performance, but the OD and OP performance is more dependent, not tracking accuracy but also the characteristics of each OD arc.
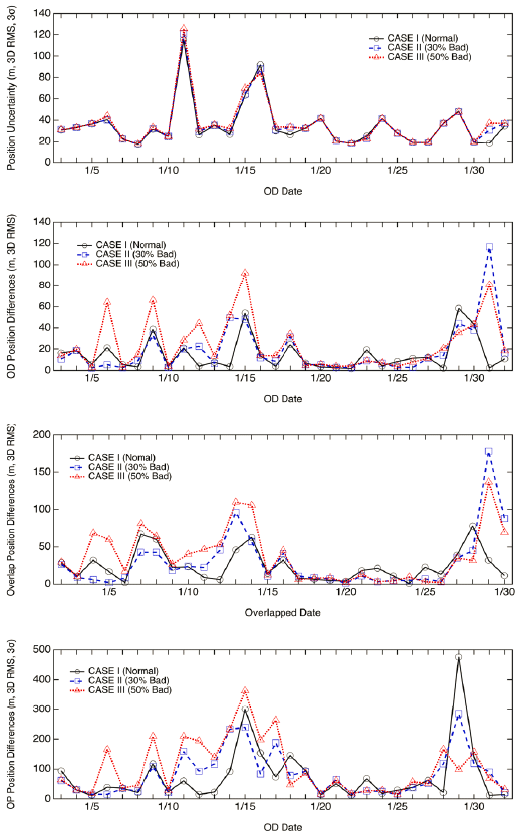
Ground stations can be faced with unexpected failure situation. In this case, ground tracking missing can be occurred and the backup plan is needed. For the examination of tracking failure effect, KPLO tracking condition configuration for 48-hr duration was assumed as follows:
-
• CASE I: No missing
-
• CASE II: KDSA 1 day missing (4 passes per 48 hours)
-
• CASE III: KDSA 2 day missing (0 pass per 48 hours)
We assumed that DSN antennas are normally operating, but KDSA has tracking miss due to sudden failure for one or two days. Normal tracking condition has four passes per day for both KDSA and DSN. The tracking condition assumption is described in Fig. 3 and Table 3. CASE I (no missing) have normal tracking condition (67% coverage for twelve lunar orbits), CASE II (1-day missing) has 4 KDSA passes per two days, CASE III (2-day missing) has no KDSA pass per two days.
In this subsection, we selected four arcs for investigation of the ground station missing effect as follows:
-
• Face-on geometry: 1/4 OD (Good PU performance), 1/16 OD (Bad PU performance)
-
• Edge-on geometry: 1/25 OD (Good PU performance), 1/11 OD (Bad PU performance)
The OD and OP performance of each arc for no missing case are shown in Table 8. Both face-on and edge-on geometries of the Earth to lunar orbit have good and bad position uncertainty performance cases. The first arc of face-on geometry is used for OD on January 4 using 48 hr tracking data (1/2–1/3), and its position uncertainty of normal tracking condition is 33.16 m. The second OD arc case (1/16) has 91.99 m of position uncertainty. For the edge-on geometry, 27.78 m and 115.55 m of are the position uncertainties for 1/25 and 1/11 OD cases, respectively. We prepared two cases for each geometry, which were relatively better and worse position uncertainties, for examining the tracking missing effect.
Fig. 7 and Table 8 show the OD and OP results for the tracking missing condition due to ground station failure. We found that the position uncertainties of all OD dates increase according to KDSA missing numbers. It is also observed that good OD precision cases (1/4 and 1/25 OD) have more performance degradation. The differences between faceon and edge-on geometries are not significant. For the OD position accuracy, the values of position differences have different trends with position uncertainty. We found that the degradation levels of face-on geometry are higher than those of edge-on geometry. Two OD arcs of edge-on (1/25 and 1/11) have no significant change of position differences performance, as shown in Table 8. For the case of OD on January 4, OD differences drastically decrease, which are ten times and seventy times worse than those of normal tracking cases for the 1-day missing and 2-day missing, respectively. We found that if tracking missing happens from 1/2 to 1/4, the OD performance cannot support the mission operation of KPLO. For the OD position differences, face-on geometry has worse performance according to tracking missing than that of edge-on geometry. For the position differences of OP, a similar trend with OD position differences are found. Faceon geometry delivers more unstable OP results than those of edge-on geometry. For OP results, it is not clearly found that the more tracking gap delivers worse OP performance. For the cases of 1/4 OD, it is shown that a 1-day missing condition gives worse OP accuracy than that of a 2-day missing condition. Because the OP accuracy is dependent on the accuracy of the OP epoch, OP performance does not have a clear trend according to the tracking gap condition. OP accuracy is also drastically degraded in face-on geometry (1/4 and 1/16). For the 1/4 OD with a 1-day tracking missing case, the OP results cannot be used for mission planning due to the enormous degradation of prediction accuracy. From the practical viewpoint, the place of the tracking gap in the arc is also essential. If the tracking gap is positioned at the starting point of OD arcs, the performance degradation becomes bigger. It is useful information for the KPLO’s mission operation and planning. As a result, we confirmed that OD performance could be worse if the tracking gap exists due to station failure or missing condition. If KPLO gets in face-on geometry, the station failure or missing is critical for the mission operation. Therefore, preparing a back-up station is an essential procedure for the successful mission operation in this period.
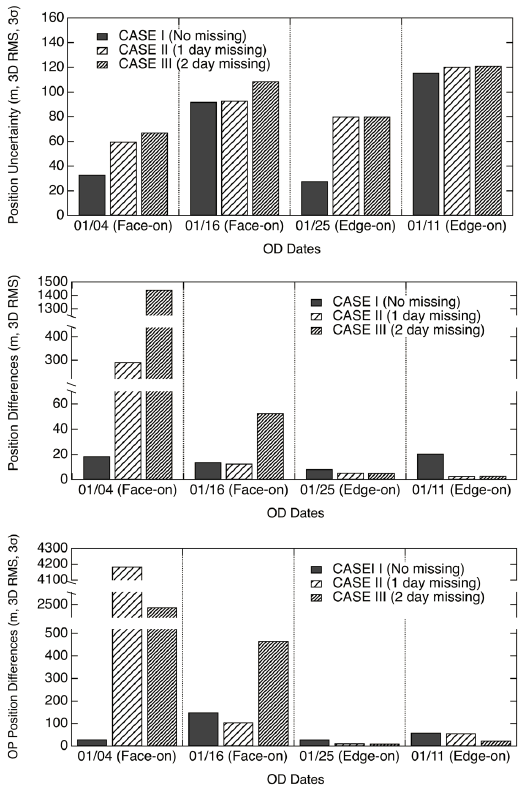
In this study, we investigated KPLO OD results for lunar mission orbit under various ground supporting conditions. The OD and OP results for the three daily tracking frequency situations, two elevation cut-off angles, three tracking accuracy cases, and tracking missing cases due to station fault were demonstrated. For daily tracking frequency effect, tracking supports more than eight passes (DSN four passes and KDSA four passes) per day is recommended for the stable orbit accuracy achievements. For the elevation cut-off angle, it is founded that a higher cut-off angle (10 deg) delivers better OD and OP performance. However, the differences between the two masking angles (5 deg and 10 deg) for tracking pass is not critical. For the tracking accuracy degradation, the OD results of 30 and 50 percent degraded measurement delivers worse performance than normal cases; however, this is not dominant to OD performance degradation. For tracking missing effect due to station failure, OD performance can worsen if tracking missing exists one or two days for two-day OD arcs. In this case, the geometry of Earth to lunar orbit affects the performance variations. Face-on geometry has more errors according to tracking missing than those of edge-on geometry. Consequently, we conclude that tracking support more than eight passes per day and ten degrees elevation cut-off angle delivers stable OD and OP performance of KPLO lunar mission orbit. If tracking accuracy is degraded about 50 percent or a tracking gap exists in two-day OD arcs, performance degradation can be huge. Therefore, a proper backup tracking plan should be prepared for the stable mission operation of KPLO in the lunar mission orbit.
5. CONCLUSIONS
In this study, ground tracking support condition effect on OD for KPLO in lunar orbit was investigated. For the stable mission operation and payload data processing, ground tracking support conditions such as daily tracking frequency, elevation cut-off angle for tracking pass limit, tracking accuracy, and tracking gap caused by station failure are critical. For the OD analysis of KPLO in lunar orbit, we used two-day OD arcs and a sequential estimation approach. For orbit accuracy assessments, position uncertainty, position differences between estimated and true orbits, and precision of overlapped arcs were used. As a result, daily tracking frequency is recommended by eight passes per day by KDSA and two different DSN antennas. Although ten degrees of angle has better OD performance, the elevation cut-off angle (5 deg and 10 deg) did not affect meaningful performance variations of OD and OP. For the tracking accuracy degradation, 30 and 50 percent bad tracking data delivered degraded OD and OP performance. In the case of station failure, one station's tracking gap can yield massive degradation of OD and OP performance in face-on geometry. Therefore, in the case of tracking missing, the backup plan is needed for the stable orbit accuracy maintenance and mission planning and operation. The ground tracking support condition should be considered for the preparation of the KPLO mission operation. This study proposes the guideline of tracking schedule configuration of the KPLO mission operation in the lunar mission phase.