1. INTRODUCTION
The majority of stars are members of binary and multiple systems; however, most investigations of stellar chemical composition do not account for stellar binarity. This is due to the assumption that the components of unevolved binary systems should have similar chemical abundance patterns. It is important to note that investigations of the chemical composition of binary components face a variety of problems that include the necessity to obtain the highest quality spectral observations and extracting the component’s spectra from the composite spectrum of the overall binary system. To understand the atmospheric chemical abundances of binary components, we need to take into account not only the standard stellar evolution theory, but also the effects that the accretion processes of interstellar and circumstellar gases, mainly hydrogen and helium, have on the stellar atmospheres.
It is proposed that accretion from the interstellar and circumstellar environment should strongly influence ob-servations of surface abundances. For example, Fowler et al. (1965) and Proffitt & Michaud (1989) pointed out the importance of mass transfer from the evolved binary companion, and Drobyshevskii (1975) and Cowley (1977) noted the importance of accretion for asteroids and planets. However, the effect of accretion processes on the surfaces of normal stars still requires further investigation. Additionally, other types of accretion processes may exist that are not yet proved or discovered. Yushchenko et al. (2015) looked for evidence that the accretion of interstellar hydrogen and helium had an effect on the atmospheres of ρ Pup (δ Sct type star) and several hundred other B-F type stars. With these effects taken into account, both Yushchenko et al. (1999) and Kang et al. (2013) presented examples of binary systems with very different abundance patterns for the components.
Herein, we present the atmospheric chemical abundances of components for the well-known eclipsing binary system, Lyn. The orbital period of this system is close to 10 days. The most recently published solutions of the radial velocity curve for RR Lyn were published by Bensch et al. (2014) and Tomkin & Fekel (2006) and the light curve solution can be found in Khaliullin et al. (2001). The masses of the binary components are determined to be 1.927 ± 0.008 and 1.507 ± 0.004 solar masses, and the radiuses are equal to 2.57 ± 0.02 and 1.59 ± 0.03 solar radiuses (Tomkin & Fekel 2006). The age of the system was estimated as 1.08 ± 0.15 billion years, and its evolutionary status is classed as before the first mass exchange (Khaliullin et al. 2001).
The chemical compositions of the components of RR Lyn have been previously investigated by Lyubimkov & Rachkovskaya (1995), and it was found that the abundance patterns of the components are different from each other based on 15 and 13 chemical elements present in the atmospheres of the primary and the secondary components, respectively.
Yushchenko et al. (2015) pointed out that this system is one of the five binary systems with a λ Boo type abundance pattern in at least one of the components, based on the criteria established by Lyubimkov & Rachkovskaya (1995). It should be noted that the total number of identified λ Boo type stars is on the order of one hundred, with no clear understanding yet of their nature. Nevertheless, for these stars, Venn & Lambert (1990, 2008) proposed a dust-gas separation mechanism, and Andrievsky (1997) regarded these stars as the descendants of close binary systems. Yushchenko et al. (2015) also suggested increasing the high temperature limit for this group to 14,000 K. Therefore, a new investigation into the abundance patterns of RR Lyn
components can be important for understanding the physical nature of λ Boo type stars. It is expected that the accretion phenomena in a binary system, such as the well-known mass exchange between components, could be responsible for the λ Boo type abundance pattern.
In this study, we aim to refine the chemical composition of the binary component using high quality spectral observations, modern software, and atomic line databases. We present the results of a spectrum synthesis fit of the observed spectrum as well as a comparison between the observed abundances of chemical elements with their second ionization potentials and the condensation temperatures of these elements.
2. SPECTROSCOPIC OBSERVATIONS AND ABUNDANCE ANALYSIS
The high-resolution spectrum of RR Lyn was obtained from the Bohyunsan Echelle Spectrograph (BOES) installed on the 1.8 m telescope at the Bohyunsan National Optical Astronomical Observatory in Korea. The signal to noise ratio (S/N) of the spectrum is higher than 150 at the center of echelle orders, the resolving power was equal to R = 82,000, and the wavelength coverage is from 3,780 Å to 9,500 Å. The observation was obtained on the Julian date 2,453,369.16 and the exposure time was 30 minutes. The extraction of the observed spectrum from the CCD image and the corresponding wavelength calibration was made using the standard IRAF software. Further data processing for the placement of continuum and the identification of spectral lines was performed using the URAN code (Yushchenko 1998).
The separation of the spectral lines for the primary and the secondary components at the moment of observation was near 135 km/s, which allowed both of the component lines to be investigated. To determine the continuum level and identify the various lines, the latest version of Kurucz (1993) SYNTHE code was used to generate the synthetic spectra of both components for the entire observed wavelength region. Line data were taken from the Kurucz (1993) database, Hirata Horaguchi (1995) line list, the VALD database (Piskunov et al. 1995), Morton (2000), Fuhr & Wises (2006), the DREAM database (Biémont et al. 2017), and other sources. Spectral lines of the appropriate quality from the observed spectrum were selected on the basis of comparison with the combined synthetic spectrum of the binary system.
To construct the combined spectrum, the synthetic spectra of both components were shifted to the observed radial velocities, broadened, and co-added. Fig. 1 shows an example of the fit obtained from the observed spectrum combined with the synthetic spectrum of the binary system. More details about the calculation procedure can be found in Yushchenko et al. (2015) and Kang et al. (2012, 2013). It is necessary to note that analyzing composite spectra is often challenging. Systematic errors can be minimized when the difference between the component spectra can be identified. In this study, we attempt to confirm or reject the large observed differences in chemical abundances between the RR Lyn binary components that were first noted by Lyubimkov & Rachkovskaya (1995).
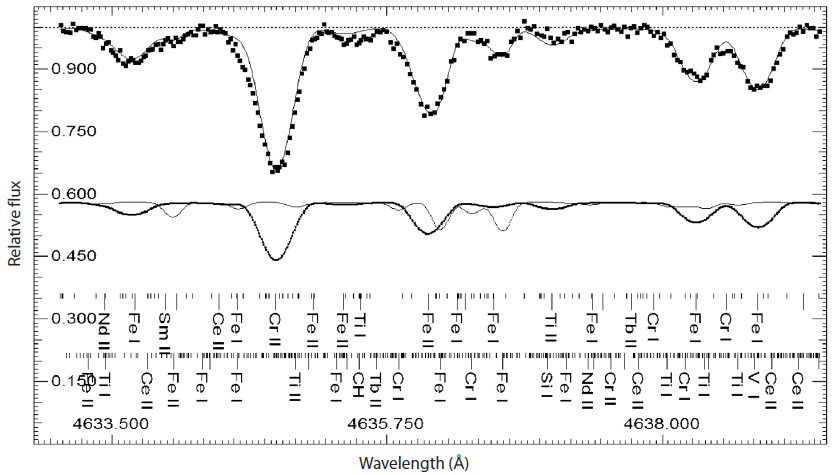
To determine the effective temperatures, surface gravities, and other parameters of the components, the method de-veloped by Yushchenko et al. (1999) and Gopka et al. (2004) was used. This method uses the iron abundances calculated for spectral lines of iron using the different atmospheric models to determine the stellar parameters. The grids of the atmospheric models with effective temperatures from 7,500 K to 8,100 K (step 10 K), surface gravities log(g) = 3.60 to 4.40 (step 0.02) for the primary component, and from 7,000 K to 7,400 K (step 10 K), surface gravities log(g) = 3.70 - 4.50 (step 0.02) for the secondary component were prepared by interpolation of the Kurucz (1993) atmospheric models. A detailed description of the method can be found in Kang et al. (2012, 2013).
The flux ratio of the binary components at wavelength λ = 5,500 Å was derived to be 3.0. For the primary and secondary components, we derived the following parameters: the effective temperatures Teff = 7,920 ± 50 and 7,210 ± 50 K, surface gravities log(g) = 3.80 ± 0.10 and 4.16 ± 0.10, microturbulent velocities vmicro = 4.60 ± 0.30 and 3.15 ± 0.30 km/s, and projected rotational velocities v sin i = 11.7 ± 1.5 and 6.1 ± 1.5 km/s. The iron abundances [Fe/H] were +0.20 ± 0.10 and -0.34 ± 0.10. The errors indicated are systematic errors associated with the method. Comparison of our values with Lyubimkov & Rachkovskaya (1995), Khaliullin et al. (2001), and Tomkin & Fekel (2006) show that higher systematic errors have been reported. Table 1 shows the parameters of the RR Lyn components published by the above authors alongside our values.
![]() |
The set of parameters shown in Table 1 were used for determining the chemical composition of both binary com-ponents. The resulting abundance calculations were then made using the spectrum synthesis method. The latest versions of Kurucz (1993) SYNTHE and Yushchenko (1998) URAN codes were used to make the fit between the observed spectrum and the combined synthetic spectrum of the binary system in semiautomatic mode.
To avoid possible errors in the oscillator strengths, the counterparts of the investigated absorption lines in the solar spectrum were found. The Liege atlas of the solar spectrum (Dellbouille et al. 1973) and the solar photosphere model (Grevesse & Sauval, 1999) were used for this purpose. It should be noted that the use of a comparison star decreases the uncertainties associated with unknown hyperfine structure and isotope shifts for spectral lines. LTE approximation calculations of the synthetic spectra were made.
Table 2 summarizes the abundance calculations. The first two columns of the table are the atomic number, the chemical symbol, and the ionization state for each of the chemical elements. In columns three to six, the number of spectral lines used and the mean atmospheric chemical abundances for both components with respect to the solar photosphere abundances can be found. In brackets after the corresponding relative abundances are the calculated error values. The last four columns of the table show the Lyubimkov & Rachkovskaya (1995) results for comparison. The numbers of used lines and relative abundances for both components are also shown.
Fig. 2 shows the abundance patterns for both components. The abundances of 34 and 17 different chemical elements were found in the atmospheres of the primary and secondary components, respectively.
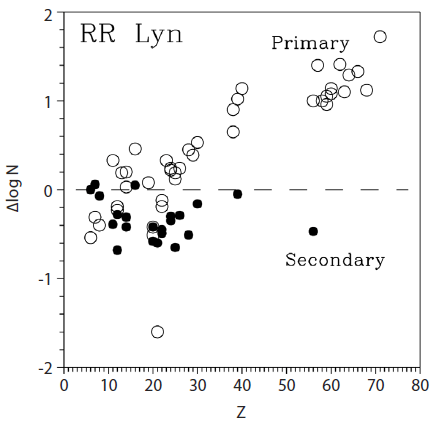
3. DISCUSSION
Table 2 and Fig. 2 clearly show that the abundance patterns for the components are significantly different from each other. This confirms the main result of Lyubimkov & Rachkovskaya (1995), which also indicated that there were large differences between the component abundances.
The primary component exhibits a relative underabundance for the CNO elements, and the solar or slightly oversolar abundances for elements with atomic numbers from Z = 11 to Z = 29. The exceptions are calcium and scandium, which are underabundant. The heavier elements, elements with atomic numbers Z > 29, are overabundant in the atmosphere of the primary. These abundance pattern features allow us to conclude that the primary component of RR Lyn is a typical metallic line (Am) star.
In contrast, for the atmosphere of the secondary component, CNO elements are close to solar abundance values. The abun-dances of other elements in the secondary are slightly under-abundant or near to solar values. This type of abundance pattern is typical for λ Boo type stars.
A possible reason for the differences in atmospheric chemical composition for both components of this binary system is that some external influences, for example the accretion of gas and dust from the circumstellar envelope, played a role in modifying what were initially similar atmospheres. The possible types of accretions that could be responsible were briefly pointed out in this manuscript and were also discussed by Yushchenko et al. (2015) in more detail.
If accretion processes occur in the RR Lyn system, it is possible to expect the dependences between the relative chemical abundances, the second ionization potentials and the condensation temperatures of the corresponding elements. Fig. 3 shows the first of these dependencies. Comparison of this figure with figure 6 in Yushchenko et al. (2015) allows us to conclude that the abundance patterns of ρ Pup and the primary component of the RR Lyn binary system have similar properties. This means that the accretion of interstellar and circumstellar gas, mainly in the form of hydrogen and helium, can change the atmospheric chemical composition of the primary component of RR Lyn as well as ρ Pup.
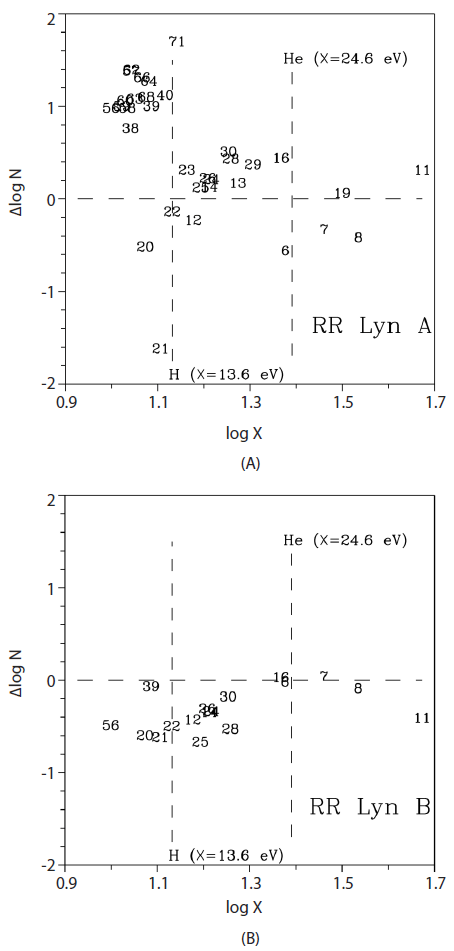
The main features of this accretion scenario were first discussed by Greenstein (1949) and later developed by Havnes (1971). It was proposed that the charge exchange reactions between hydrogen atoms from the interstellar medium with atoms from the stellar photosphere results in a decrease of the relative abundances of elements with second ionization potentials close to the ionization potential of hydrogen, namely those close to 13.6 eV. The result of possible resonance in the charge exchange reactions is that the escape velocities of atoms with the above highlighted second ionization potentials, and perhaps the production of cosmic rays, would be able to brake the stellar rotational velocities. Böhm-Vitense (2006) found that a similar effect could also be important for helium atoms.
The abundance pattern of the secondary component of the Lyn binary system includes only 17 chemical elements, but the increase in the relative abundance of chemical elements with second ionization potentials between 13.6 and 24.6 eV (the ionization potentials of hydrogen and helium) are clearly detected in the bottom panel of Fig. 3. It is therefore possible that the accretion of hydrogen and helium from outer space can be an important process for both components of the RR Lyn binary system.
Fig. 4 shows a plot of the relative chemical abundances in the atmospheres of RR Lyn components against the condensation temperatures of the corresponding elements. The negative correlation illustrated by this plot is typical for λ Boo type stars (Venn & Lambert 1990, 2008). The correlation can be explained by a dust-gas separation mechanism resulting from accretion in a circumstellar cloud. Elements with higher condensation temperatures condense into dust particles in regions with higher temperatures, which are closer to the stellar surface. After condensation, the dust particles are influenced by solar radiation pressure and thus are effectively removed from the circumstellar environment. This explanation is one of the most plausible to justify the negative correlation observed between the relative abundances and condensation temperatures in the atmospheres of λ Boo type stars.
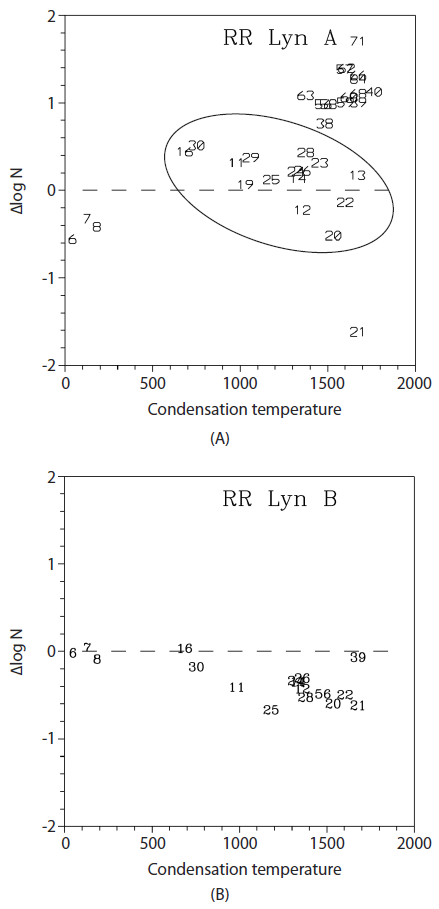
Fig. 4 shows that a negative trend is evident for the data presented in the bottom panel of the figure. The correlation coefficient is equal to -0.73 +/- 0.17. This implies that the dust-gas separation mechanism proposed by Venn & Lambert (1990, 2008) can explain the observed distribution for the relative abundances in the atmosphere of the secondary component.
Further discussion is needed for the main component shown in the upper panel of Fig. 4. Elements with condensation temperatures higher than 1,000 K and elements with relative abundances higher than that of strontium (Z = 38) are not usually detected in the spectra of λ Boo type stars. For CNO elements and scandium (second ionization potential = 12.8 eV), the underabundances of these elements can be explained by charge-exchange reactions with hydrogen.
The remaining elements, namely the 15 elements located in the highlighted elliptical area of the upper panel of Fig. 4, also show a strong negative correlation. The correlation coef-ficient is -0.57 +/- 0.17. This evidence gives strength to the conclusion that the dust-gas separation scenario could be the most important influencing process for these elements while the other elements are strongly influenced by other physical effects.
Figs. 3 and 4 allow us to surmise that the different accretion scenarios presented do take place in the RR Lyn binary system. It is worth taking into consideration that the abundance patterns of both components can be the result of a mass transfer process between the two components. The influence of mass transfer on the distribution of the relative abundance in the binary components was not investigated for heavy elements earler.
Finally, we confirm the large chemical composition differences observed for the RR Lyn binary components first published by Lyubimkov & Rachkovskaya (1995). We assert that the atmospheres of these components are strongly influenced by different types of accretion. These are the accretion of hydrogen and helium from interstellar or circumstellar gas (Greenstein 1949;Havnes 1971;Böhm-Vitense 2006), and dust-gas separation (Venn & Lambert 1990, 2008). It is necessary to point out that the net metallicity difference between the two components is significant and that further investigations may be needed to explain this difference in relation to accretion effects. Additionally, the diffusion effects first proposed by Michaud (1970) should be also taken into account during further studies.
More careful analyses of RR Lyn and similar binary systems can help us to understand the physical processes that occur in stellar atmospheres. The theory of accretion effects due to hydrogen and helium from the interstellar or circumstellar environment on the atmospheres of normal stars was proposed by Greenstein (1949) and Havnes (1971), but this scenario had very few observational confirmations until now with the exception of Böhm-Vitense (2006) and Yushchenko et al. (2015). The detailed theory of this effect has not yet been fully developed, and would require new observational results and further research.