1 INTRODUCTION
The characteristics of the CubeSat are defined by Stanford University and California Polytechnic State University. The standard 1-U size is a 10 cm cube of very low weight (1 kg or less) (Puig-Suari et al. 2001). Developing a CubeSat represents an increase in the opportunities to explore space in fast development of technology. The development of CubeSat is a good foundation for education and the design of space research instruments in the future. The planned launches of CubeSats demonstrate that interest in satellites is growing. CubeSats have various mission types including military use, intelligence services, geospace research, atmospheric research, ionospheric plasma density, and electric field measurement (Straub 2012).
Communications satellites are an essential part of spacebased activities such as receiving data from satellites and in-orbit operations (Toyoshima et al. 2005). People are interested in the payload of the spacecraft, which accounts for part of the funding. However, if the communication systems malfunction, then the payload will be useless in space (Klofas & Leveque 2013).
A satellite communication system consists of a space segment, a control segment, and a ground segment. Satellites are involved in the space segment as a communication system. The control segment and the ground segment contain all equipment to control and monitor the satellites and earth stations (Maral et al. 2009). In this paper, the communication system is classified as a satellite, which is the space segment, and a ground station, which contains the control segment and ground segment.
According to a survey of CubeSat communication systems in the last five years, most of the CubeSats employed very high frequency (VHF) band, ultra high frequency (UHF) band, S band, or X band frequencies (Klofas 2016). In addition, data rate and modulation depended on the mission type or frequencies of satellites. A number of CubeSats communicate in 1,200 bps or 9,600 bps, and a few in Mbps.
Scientific CubeSat with Instruments for Global Magnetic Fields and Radiations (SIGMA), KHUSAT-3, is a 3-U CubeSat developed by Kyung Hee University (KHU) with international collaboration. It is planned to launch in the first quarter of 2017, and its launch vehicle is SpaceX, Falcon 9. SIGMA has two payloads: Tissue Equivalent Proportional Counter (TEPC) and a Miniaturized Fluxgate Magnetometer (MAG). TEPC, the primary payload, measures the linear energy transfer (LET) spectra and investigates the equivalent dose for radiation fields in space environments (Nam et al. 2015). MAG is the secondary payload. It measures ultra low frequency (ULF) waves and has a resolution of 0.1 nT.
SIGMA employs the VHF band for uplink and the UHF band for downlink. This paper presents the communication system of SIGMA and a telecommunication test to verify its reliable communication between the CubeSat and the ground station.
For this study, we carried out a link budget analysis and communication tests, and confirmed the reliability of communications. We also compared the field test results with the link budget calculation, and discussed the problems of the communication system.
2 COMMUNICATION SYSTEM OF SIGMA
CubeSat has a transceiver and an antenna module that are connected to the on board computer (OBC). Fig. 1 shows the flight model of SIGMA. The CubeSat transmits various data sets that have a beacon signal, housekeeping data, and the payload data. These downlink signals are modulated by 9,600 bps scrambled binary phase shift keying (BPSK). In addition, the CubeSat receives commands modulated by 1,200 bps audio frequency shift keying (AFSK) from the ground station. The data rate is determined by the length of commands in uplink and the data size of payloads in downlink. All data and commands follow the AX.25 protocol. The communication radio band frequency employs VHF band 145.210 MHz for uplink and UHF band 435.780 MHz for downlink. These frequencies are within the amateur band. Fig. 2 shows an overview block diagram of the SIGMA communication system. Its specifications are listed in Table 1.
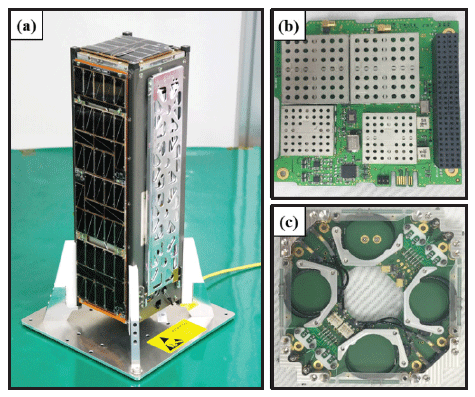
The transceiver module of SIGMA is the TRXVU from Innovative Solutions In Space (ISIS; Delft, Netherlands), which is assembled on top of the avionics stack. This full-duplex transceiver module is designed for CubeSat applications. The transmitting data modulation supports the BPSK modulation scheme. The scrambling polynomial of BPSK modulation is 1+X12+X17, G3RUH scrambling. The transceiver also receives commands from the ground station. There is no scrambling in AFSK modulation.
The antenna module of ISIS is connected to the transceiver with RG 178 coaxial cables. The antenna system is deployed by using I2C commands. A dipole antenna performs radio telecommunication with two symmetrical radiating arms. The total length of the antenna for each band is a half wavelength of carrier waves, and a feed point is at the center. This type of antenna is a basic form of all antenna types and is commonly used for the HF band (Lim et al. 2009). The CubeSat antenna module is mounted in the top chassis of the CubeSat and deployed toward each direction. Four whip antennas are rolled up and stowed inside of the housing. Burn wires made of Dyneema hold each rolled-up antenna. The antennas are deployed when the wires burn.
A ground station consists of VHF and UHF Yagi antennas, a satellite tracking rotator, a transmitting section, and a receiving section. The ground station contains equipment for a terrestrial network interface, monitoring, and electrical connections. The ground station handles various subsystems and performs the satellite communication.
The main equipment has a terminal node controller (TNC), transceivers, a receiver, and an antenna subsystem. A personal computer (PC) controls the G-5500 rotator by a rotator controller (GS232B) from Kenwood (Tokyo, Japan). The antenna automatically tracks the satellite using tracking software. For transmitting commands, we use the Kenwood TS-790A and TS-2000. One of these is redundant equipment. The commands from the PC are sent to a TNC-X from Coastal ChipWorks (Fredonia, U.S.A.). A FUNcube Dongle Pro+ from Hanlincrest Ltd. (London, U.K.) connected to the UHF Yagi antenna is used only to receive data from the CubeSat. For redundancy, a USRP B200 from Ettus Research (Santa Clara, U.S.A.) is able to transmit and receive data as well; however, in case of a transition, an amplifier is required. The USRP is mainly used within a short distance of the laboratory. The RF equipment for the KHU Ground Station is shown in Fig. 3.
Most of the communication software products using in SIGMA are freeware; few computer programs were specifically designed for SIGMA. First, Ham Radio Deluxe (HRD) version 5.0 is software for satellite tracking and controlling the rotator and the transceiver. For transmitting commands, Commander, which is used for transmitting commands to CubeSat, is coded in the C language and was developed by the SIGMA team.
To receive data from the CubeSat, we use three software packages: SDR Sharp, Soundmodem, and Telemetry Monitoring Program (TMP). SDR Sharp is PC-based Software Defined Radio made by Youssef Touil. This software generates down converted sound wave data. Soundmodem is a dual-port Packet-Radio TNC software developed by Andrei (UZ7HO). This freeware version did not include scrambled modulation; therefore, Andrei developed a SIGMA version that decodes scrambled BPSK data. Fig. 4 shows the beacon data of SIGMA in SDR Sharp and Soundmodem. Using freeware, we developed a TMP coded in Java, which allows us to confirm received data using TMP in real time. This is shown in Fig. 5.
3 LINK BUDGET ANALYSIS
In analyzing satellite communication, the variables for the link can be explained by the signal-to-noise ratio (S/N) for analog communications and the bit error rate (BER) for digital communications. The carrier-to-noise ratio (C/N) is a basic variable representing both analog and digital communications. It is calculated by using the link budget. In the case of digital communications, we can express the link performance as a numerical value of the BER by converting C/N to energy per bit to noise spectral density ratio (Eb/N0) (Lee 2013). Eb/N0 depends on the modulation and determines the bit-error probability (Moon et al. 2004). Satellite communication systems are designed for a link margin of 3–8 dB (Yoon 2012).
Table 2 lists the input parameters used to calculate the link budget based on the ground station system at KHAO and the transceiver and antenna module of the CubeSat. The specifications for each communication system and the average value of the loss were used in the calculations. We calculated the link margin as shown in Table 3 by using parameters listed in Table 2. Both the link margin of the uplink and downlink exceeded 3 dB, which is the threshold for reliable communication. This means that communications between the CubeSat and the ground station are theoretically possible.
4 COMMUNICATION TEST & RESULTS
We carried out two tests of radio telecommunication between CubeSat and a ground station. The first test was an indoor laboratory test. We transmitted commands and received beacon signals from the CubeSat using the ground station equipment. Second, in a far-field test, we checked the basic data communication using the ground station equipment. In addition, we added several parameters, such as space loss and antenna pointing, that were considered for the space operational environment.
The LAB test was carried out indoors. In an initial performance test, we checked the data communication using USRP, which is used for subsidiary equipment in the ground station.
We tested the transmitting and receiving data-packet communication controlled by the PC without environmental variables. For the CubeSat part, the transceiver and antenna module were connected to the PC through the OBC. For the ground station area, we used a PC, TNC, transceiver (TS-790A), FUNcube Dongle Pro+, and a small portable antenna (since the UHF Yagi antenna has high gain at a short distance).
In the uplink test, we checked for status changes in CubeSat after transmitting commands from the ground station. In the downlink test, beacon signals from CubeSat were checked. At the ground station, decoded beacon data were displayed by SDR Sharp and Soundmodem.
In the LAB test, there were no issues. We confirmed the reliability of the SIGMA communication system in the nearfield. The test environment is shown in Fig. 6.
According to the results of the far-field distance calculations, we must conduct the far-field test at a distance of more than 104.59 m away from the ground station. Therefore, the farfield test was carried out on the rooftop of the building at a distance of 0.4 km. In addition, we carried out the far-field test at a distance of 8.7 km because the test location at 0.4 km was lower than the position of the ground station antenna. The test location at a distance of 8.7 km was a mountain that had a higher elevation than the ground station antenna.
In both the uplink and downlink tests, the test variable parameters are considered for free space path loss, Doppler frequency shift, antenna status, and satellite body attitude.
The equipment for the test was the same as that in the LAB test, except for the VHF and UHF Yagi antennas of the ground station. Fig. 7 shows the straight-line distance between CubeSat and the ground station in the far-field tests.
The radio signal strength transmitted by an antenna has different properties depending on the distance. The signal distribution is not uniform in the near-field but is omnidirectional in the far-field. There are three divided regions surrounding an antenna: reactive near-field, radiating near-field, and far-field regions. The reactive near-field region is where the reactive fields predominate. The boundary distance is from the antenna surface. The radiating near-field region is the distance , where the radiations fields predominate. is the wavelengh, and D is the largest cross-sectional diameter or dimension of the antenna.
The distance of the far-field region is R > 2D2/λ, which is independent of the distance in the distribution of an angular field (Balanis 2005). We considered the near-field as a distance of the reactive near-field. The far-field distance was assumed to be the maximum result (104.59 m), which is the far-field distance boundary of the UHF ground station antenna. Table 4 lists the calculation results for the far-field distance. We carried out the far-field tests at a distance of more than 104.59 m away from the ground station.
FSPL is the ratio of the powers for receipt and transmission between isotropic antennas (Maral et al. 2009). It is usually expressed in dB and refers to the frequencies and distance. The equation is(1)
where d is the distance from the transmitting antenna in kilometers, and f is frequency of the signal in megahertz (Maini & Agrawal 2007). The calculation results depending on the expected orbit of SIGMA are listed in Table 5.
This test for uplink used attenuation to approximate the free space path loss. According to the results for an altitude of 720 km in Table 5, Cubesat has to recieve commands at an additional attenuation of more than 65 dB at a distance of 0.4 km, and 40 dB at a distance of 8.7 km from the ground station. For this test, attenuators were added to the ground station transceiver. The transceiver had a frequency of 145.210 MHz, and the maximum transmission power was up to 50 W. CubeSat received all commands up to an attenuation of 117.78 dB, but CubeSat only partially received at 122.78 dB, and did not receive at all at 132.78 dB. In the downlink test, attenuators were added to a side of the FUNcube Dongle, and we successfully received all data up to 162.33 dB. The results are listed in Table 6.
We carried out the uplink test at a distance of 8.7 km. As with the test at a distance of 0.4 km, CubeSat did not receive the commands with lower attenuation than the requirement. The ground station received data from the CubeSat up to an attenuation of 159.08 dB. Table 7 lists the results. The results of the uplink test is short of the 132.84 dB attenuation that is required at an altitude of 720 km. If the uplink transmitting power is increased to 90 W, the CubeSat will receive commands at higher attenuation.
The attitude of CubeSat can affect communication performance since the CubeSat VHF and UHF antenna directions are different from each other. Before antenna deployment, the whip antennas are rolled up and stowed inside the module. If the antennas fail to deploy, it will seriously affect radio communications.
The test results depending on antenna deployment at a distance of 0.4 km are listed in Table 8. When the antenna deployed to one side only, CubeSat received all commands. When the antenna was not deployed, CubeSat received partial commands. It was expected that CubeSat would not receive commands when the antenna was not deployed.
Variable | Status | Commands or Data |
---|---|---|
CubeSat | Not deployed | Partially received |
Antenna Deployment | Deployed one side only | Received |
|
||
CubeSat Attitude | 0° | Received |
30° | Received | |
60° | Received | |
90° | Received |
The variable parameters for the downlink test were the same as those for the uplink test. As with the uplink test, the FUNcube Dongle received partial data when the antenna deployed to one side only. However, if the CubeSat was placed at a greater distance without antenna deployment, it was unable to communicate with the ground station. The received signal strength indication (RSSI) value of the CubeSat decreased by approximately 30 dB compared with the status when the antenna was deployed.
The lower portion of Table 8 lists results for the satellite body attitude. CubeSat tried to receive commands between 0° and 90° on the z-axis of the CubeSat basis (Fig. 8). As a result, all commands and data were received at every attitude of CubeSat.
Table 9 lists the results at a distance of 8.7 km from the ground station. In this test, a 35 dB attenuator was added to the Yagi antenna of the ground station. We changed the direction angle of the ground station antenna within 82° (uplink) and 60° (downlink) in our test. Then, we tried to rotate the antenna until it was unable to receive commands or data. As a result, the communication allowance range was ±40° for the uplink VHF and ±5° for the downlink UHF (Fig. 9). The UHF Yagi antenna was less affected by the environment around the ground station since the boom length of the UHF Yagi antenna was longer than that of the VHF Yagi antenna.
In addition, we changed the CubeSat attitude from -90° to +90°, as shown in Table 9 and Fig. 8. The uplink results are different from the downlink results. This difference depends on the position of the CubeSat VHF and UHF antennas.
A Doppler frequency shift was observed between the satellite around the earth and a ground station that communicates with the moving satellite (Lim et al. 2009). The calculated Doppler frequency shift range is 145.206 MHz to 145.214 MHz for uplink, and 435.769 MHz to 435.791 MHz for downlink at an altitude of 720 km. Therefore, the test frequency range is determined by using the Doppler frequency shift range. We controlled the uplink frequency using a transceiver at the ground station, and transmitted commands 30 times in 30 seconds. SDR Sharp connected to the FUNcube Dongle controlled the downlink frequency in units of 1 kHz. Then, we checked that all commands from the ground station were received and were the same data from CubeSat. Table 10 lists the results based on the Doppler frequency shift.
In the test at a distance of 8.7 km, instead of considering a specific frequency range, we changed the frequencies of the ground station until the commands or data were received. An attenuation of 35 dB was adopted for the ground station. The transmitting power of the uplink was 50 W.
Far-field tests were carried out under various conditions such as variable attenuation, antenna deployment status, CubeSat attitude, and Doppler frequency shift. There was an issue with the uplink process in the 0.4 km test environment. The CubeSat did not receive the commands from the ground station at 55 dB attenuation, but the ground station received all signals from the CubeSat.
In the 0.4 km uplink communication, we found that the main reason for this issue is the far-field test environment around KHAO. A dome structure behind the Yagi antenna interfered with the uplink signals, and there was signal interference in the same frequency band around the ground station. The average intensity of the transmitting signal attenuates because the walls of buildings generate an increasing number of multi-reflections (Blaunstein & Levin 1996).
In addition, there is significant vegetation covering the mountain around the Yagi antenna direction toward the farfield test spot. Propagating signals in an environment that has many trees can cause multiple scattering, diffraction, and absorption of signals since many discrete scatterers (such as randomly distributed leaves and branches) form a random medium (Meng et al. 2009).
However, during the 8.7 km far-field test, we did not experience any problems. Furthermore, there are no issues in our voice communication with OSCAR. Comparing the analysis and test results, we confirmed that the communication system can sufficiently communicate between SIGMA and the ground station.
5 CONCLUSION
SIGMA will be launched on Q1 2017 loaded in an American launch vehicle, Falcon 9. The flight model of SIGMA is ready to launch. The center frequency for uplink is 145.210 MHz, and commands are transmitted using 1,200 bps AFSK signals. The downlink frequency is 435.780 MHz, and data is received from the CubeSat using 9600-bps scrambled BPSK signals. A ground station of SIGMA was constructed in KHAO, and it controls the CubeSat and receives data. By using HRD, we can predict a contact time with the satellite and control the direction of the ground station antennas depending on the orbit of the satellite. We use Commander to transmit commands with a simple click. In addition, a FUNcube Dongle Pro+ connected to the UHF Yagi antenna is used with SDR Sharp and Soundmodem for receiving data. The status of SIGMA is checked in real time by TMP.
Before operating the CubeSat in orbit, we carried out tests for reliable telecommunication between the CubeSat and the ground station. In the LAB test, all attempts at communication were successful. In addition, in a far-field test, variables were considered for the space environment. Each of the far-field tests was measured at a distance of 0.4 km and 8.7 km from the ground station. For satellite body attitude, CubeSat antenna deployment, and Doppler frequency range, the test results showed normal values; however, we had one issue with the uplink test that had a 65-dB attenuation at a distance of 0.4 km. This test had an insufficient result compared with the theoretical values in the link budget analysis.
We realized that the CubeSat could not receive uplink commands in the test environment surrounding KHAO. The mountain and the dome structure around the Yagi antenna affected the transmitting signal on the groundbased test field. Furthermore, signal interference in the same frequency band affected the transmission of signals. However, we confirmed that the ground station has sufficient performance by a space-based test with OSCAR.
Our test method and analysis are practical approaches for CubeSat communication tests. From our results, a more precise measurement of the communications can be performed. This should be useful in designing a low-cost CubeSat communication system.