1. INTRODUCTION
Recently, the need for precise measurement for aviation radiation exposure is increasing. The radiation fields in aircraft altitude are quite complex, consisting of several different particles such as proton, neutron, muon, pion, photon, and electron. Moreover, those vary with latitude, longitude, and altitude. The ranges of radiation exposure at aviation altitudes are also much larger than are found in most other radiation protection areas on the ground. The only instrument for the complete measurement of the cosmic mixed-radiation field is a tissue-equivalent proportional counter (TEPC; Lindborg et al. 1999). The TEPC dosimeter of KASI has been developed to measure the radiation exposure in various space radiation environments (Nam et al. 2015;Malimban et al. 2019). Also, the LEO-DOS (the low earth orbit space radiation dosimeter) is being developed as a scientific payload of the NEXTSat-2, one of the next-generation small satellite programs in Korea launched in 2022.
In this paper, we report the development process of a practical space radiation dosimeter for aviation safety (SRDAS) for a radiation exposure monitor for aircrew and passengers. We also report some preliminary results of the verification of the SRDAS instrument for the flight experiment over the Incheon airport (ICN)- John F. Kennedy airport (JFK) air route. The primary purpose of this experiment was to verify the performance and usability of our SRDAS instrument. The equipment used during the flights consisted of two SRDAS and two silicon sensor detectors (Liulin), and Liulin detectors are used as benchmark instruments.
2. SPACE RADIATION DOSIMETER FOR AVIATION RADIATION
A TEPC is known to be a standard instrument for measurements in a mixed radiation field. Particularly in aircrew radiation dosimetry, the TEPC is of major interest for use as a reference instrument (Lindborg et al., 1999;Rollet et al., 2004). It measures the microdosimetric distribution d (y) of an absorbed dose as a function of the lineal energy y over up to five orders of magnitude. Dose equivalent is calculated by folding this distribution with the quality factor as a function of linear energy transfer (LET), as defined in ICRP74. Fig. 1 shows the SRDAS-TEPC. Fig. 1(a) is the complete assembly of SRDAS. The electronics box is located below the TEPC chamber. The shape of TEPC shown in Fig. 1(b) is a 6 cm inner diameter sphere. Since the TEPC is filled with a pure propane gas at low pressure (13.9 torrs), it simulates a tissue volume with a diameter of 2 μm. The sphere's wall is made of a tissue-equivalent plastic (A-150) with a thickness of 3 mm. The TEPC sphere is contained in a cylindrical aluminum structure of 1.5 mm thickness and the required electronics such as the charge sensitive preamplifier. The complete assembly is cased inside a portable trolley with dimensions of an aircraft hand-baggage to minimize microphonic noise caused by cathode vibration problems, which may occur during a real-time measurement experiment inside the airplane. Table 1 lists the technical design specifications of SRDAS-TEPC.
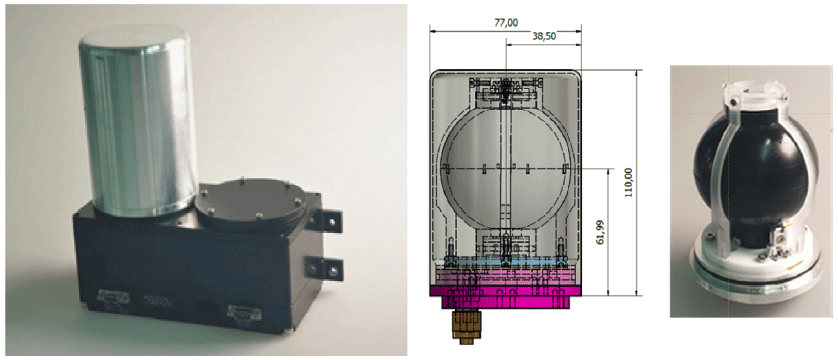
The block diagram of the SRDAS electronics system is shown in fig. 2. The electronics package of the SRDAS instrument consists of four interconnected printed circuit boards: TEPC-AMP board, APP board, LVPS board, and POWER-INTF board, which stand for the TEPC amplifier board, the analog pulse processor board, the low voltage power supply board, and the power interface board, respectively. The TEPC-AMP board is used to interface the charge sensitive preamplifier, located inside the TEPC chamber. The charges produced by individual energy deposition events in TEPC are converted to a voltage pulse via a preamplifier. The purpose of TEPC-AMP is to transform the shape of the event pulse and filter out the noise from the signal of interest. The pulse shaping circuitry consists of two Sallen-Key filters to meet this requirement, providing four poles of integration and a signal gain. The output pulse of TEPC-AMP has a shaping time of 2 μs, an output pulse width of 4.7 μs. These functions were implemented using a low cost, commercial, operational amplifier AD8034. TEPC-AMP has two gain outputs. The ratio between a low-gain and a high-gain is 20:1, which will cover the lineal energy range of 2–1,000 keV/μm. TEPC-AMP contains a negative high voltage supplier (APN-08-604). The voltage level is typically set at around −510 V. In the APP board, the two amplifier outputs are conditioned using two peak-holds and an analog multiplexer (MUX) and converted to digital information using single analog-to-digital converter (ADC) and a microcontroller (MSP432P401R, mixed-signal microcontroller) to implement a 512-channel multi-channel analyzer, which will record a low-LET and a high-LET linear energy spectrum. It also contains SD CARD to storage data, RTC (Real-time clock), and RS232 communications chip. The LVPS provides a low noise power supplies 3.3 V for a digital electronics system, –5.5 V and +5.5 V for an analog electronics system. The POWER-INTF delivers DC power to LVPS and functions as a switch for the SRDAS-system. SRDAS system operates with a 7.4 V Li-ion battery, which requires DC-12V for charging.
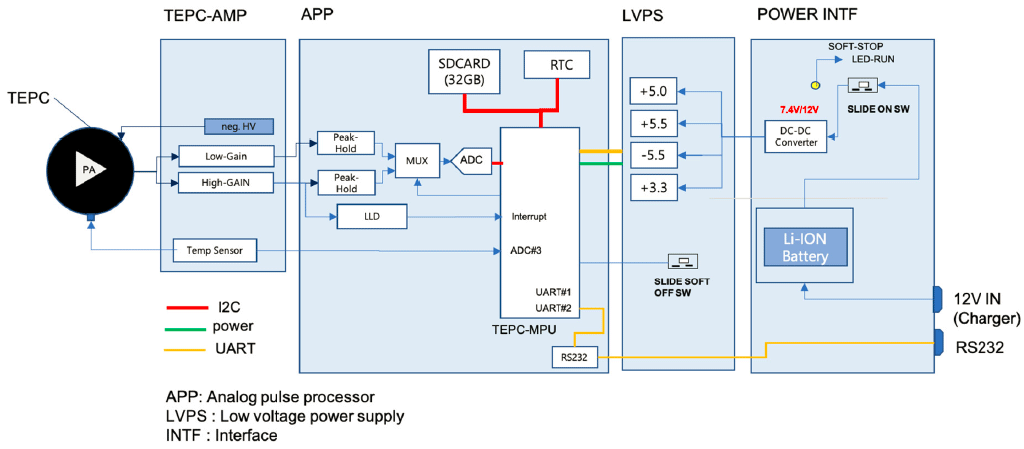
The quantity obtained from TEPC is the lineal energy (y), which is defined as the quotient of the energy imparted (ε) in a volume due to a single energy deposition event and the mean chord length (I) of that volume (Booz et al. 1983). The lineal energy is the stochastic analog of the LET and is expressed in keV/μm. Using lineal energy, we can easily obtain an absorbed dose and derive a mean quality radiation factor to calculate the dose equivalent in a mixed radiation field. Fig. 3 shows the algorithm for the dose calculation of TEPC. Since the TEPC was equipped with an internal alpha calibration source, a self-calibration of the raw pulse height spectra can be performed. From the calibrated linear energy spectrum, microdosimetric parameters such as a mean the quality factor (Q), a frequency mean lineal energy (yF), a dose mean lineal energy (yD) are calculated (ICRU report 36, Booz et al. 1983). The absorbed doses (D) for both irradiation conditions were also calculated as follows.
Then, the dose equivalent (H) was subsequently derived as follows
where Q is the average quality factor derived from the given expression.
In practice, Q(yi) is assumed to be equal to the quality factor Q(L) given in ICRP60 (ICRP 1991). It has been introduced to weigh the absorbed dose for the biological effectiveness of the charged particles since the effects caused by radiation depend not only on absorbed dose but also on radiation type (i.e., ionization density). The quality factor at a point in tissue is given by table 2 as follows.
yi (keV/μm) | The quality factor, Q(yi) |
---|---|
yi ≤ 10 | 1 |
10 < yi < 100 | 0.32L − 2.2 |
yi ≥ 100 | 300/ |
Since TEPC measures energy deposition in its gas, an additional calibration is needed to express an instrument response in terms of ICRU operational quantity, the ambient dose equivalent, H*(10). The total ambient dose equivalent is given by the sum of a low-LET and a high-LET component. These components are defined as the contributions below, and above ten keV/μm of the lineal energy scale and are calibrated by Klow and Khigh factors (Latocha 2017).
In this dose calculation algorithm procedure, the statistical uncertainties are calculated as follows (Aslam et al. 2003).
In this study, the absorbed dose, the quality factor, and the dose equivalent were evaluated from the linear energy spectrum measured with TEPC according to the procedure in Fig. 3.
3. Results and Discussion
The SRDAS-TEPC contains an internal alpha source (i.e., 241Am), which can be used for the lineal energy channel calibration. The peak ADC channel for 5.5 MeV alpha particle of 241Am represents 150 keV/μm in lineal energy. If the alpha peak is shifted from the preferred ADC channel, a correction must be done in high voltage adjustment to the detector, changing the gas gain. After the initial calibration, the detector performance did not change significantly for several months during the whole flight measurement test. The performance verification and calibration of SRDASTEPC for 252Cf radiation were conducted at the Korea Research Institute of Standards and Science (KRISS). From the lineal energy measurement for the net 252Cf neutron field with TEPC, the frequency-mean and dose-mean lineal energy was evaluated to be 36.49 keV/μm and 72.52 keV/ μm for the neutron-only area, respectively. These values are consistent in general as shown int Table 3. Therefore, the SRDAS-TEPC can be successfully used in the neutron monitoring and dose measurement in a practical radiation protection purpose by measuring an absorbed dose of the neutron as well as evaluating the mean quality factor of the neutron from the lineal energy measurement of the neutron in the mixed radiation field.
yF (keV/μm) | yD (keV/μm) | Q | |
---|---|---|---|
SRDAS-TEPC | 36.49 | 72.52 | 13.98 |
Reference (Chang & Kim 2008) | 38.80 | 73.90 | 17.40 |
The flight measurement with the SRDAS instrument was conducted on the round-trip flight between ICN and JFK. We also used the Liulin semiconductor detector to cross-validate with SRDAS measurements. For this verification experiment, a total of four instruments were used. Those are two Liulin detectors (Liulin-MDU2 and Liulin-MDU3) and two SRDASs (SRDAS-101A and SRDAS-103). A Liulin instrument for the experiment was manufactured at the Space Research and Technology Institute of the Bulgarian Academy of Sciences in Sofia (Dachev et al. 2009). Liulin semiconductor silicon detectors measure charged particles' energy deposition in the charge sensitive region of a Hamamatsu S2744-08 positive intrinsic negative photodiode (PIN-diode) with a thickness of approximately 300 μm and a mass of 0.16597 g. Fig. 4 shows flight dose profiles measured for ICN-JFK flights on 16 November and 19 November 2019. Flight data were taken every minute while the plot shows 5-min averaged data. The total accumulated dose for the ICN-JFK route is 72.83 μSv for SRDAS TEPC and 100 μSv for Liulin, respectively. Similarly, for the JFK-ICN route, the total dose is 85.12 μSv for SRDAS and 105 μSv for Liulin, respectively. The flight dose profiles on both SRDAS and Liulin showed the similar patterns. The dose rate shows somewhat larger fluctuation in Fig. 4. This is not a shortcoming of TEPC, but a unique characteristic of TEPC. TEPC demonstrates that the real energy fluctuations that the particles really deposit in the detector. At the aircraft altitudes, there are considerable neutrons causing a high-LET and they contribute to higher dose values even though the flux is small. As TEPC is more sensitive to the high-LET than Liulin, the measured dose’s fluctuation becomes larger than Liulin. We modify the Fig. 4 to show this more clearly. And the uncertainty of TEPC measurements is less than 30% which is known to be acceptable criteria as a public dosimeter.
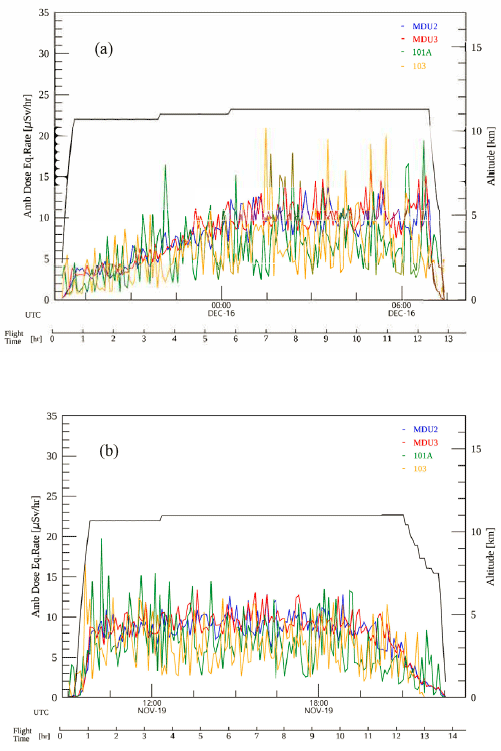
More detailed results are shown in Table 4. Dose rates and ambient dose equivalent rates were compared at vertical cutoff rigidity, 1 < Rc < 4 GV and GV is the unit of the rigidity. For the flight altitudes, the values correspond to the flight interval between 00:08 UT and 06:08 UT for the ICN-NY route and flight interval between 09:55 UT and 18:56 UT for JKC-ICN route, respectively. For the ICN-JFK route, the ambient dose equivalent rate, dH*(10)/dt from two SRDAS’s were 7.40 ± 3.59 μSv/h, 7.83 ± 4.45 μSv/h, respectively. The deviation between the two SRDAS’s was 1.55%. On the other side, the ambient dose equivalent rate, dH*(10)/dt from two Liulins (MDU2 and MDU3) were 10.06 ± 1.42 μSv/h, 10.64 ± 1.94 μSv/h, respectively. The deviation between two Liulin- MDUs was 6.2%. Dose responses of Liulins are substantially higher than those of TEPCs. It should be noted that the larger over-response of Liulin-MDUs is well consistent with the previous experiments (Meier et al. 2016;Straume et al. 2016). During the calibration of Liulin, ground-based exposure to 137Cs gamma rays showed more than 10% overestimation (Dachev et al. 2002), which indicates that the Liulin-MDU instrument has a substantial over-response to low LET radiation. Also, the very low sensitivity of Liulin- MDU silicon detectors to neutrons, which contribute significantly to the dose rate at aviation altitudes, has to be considered (Straume et al. 2016).
Silicon detectors can nevertheless be used to measure the dose rate at aviation altitudes if a relevant conversion factor Cfield is determined beforehand with a calibrated reference instrument to measure H*(10), e.g., a TEPC (Meier et al. 2016). Cfield is the proportional factor between the absorbed dose in silicon and the ambient dose equivalent of TEPC:
Measured Cfield from this experiment was 2.68 for the ICNJFK route, 2.83 for JFK-ICN route, respectively. Comparing with the reference results (See Table 2 in Meier et al. 2016), Cfield = 3.1 ± 0.4 at the region RC 4.1. Our observations are reasonably consistent with previous reports. The Cfield would be a critical parameter for the flight experiments.
4. CONCLUSION
We developed and verified the SRDAS instrument for aviation radiation dosimetry to secure the aircrew and passengers from space radiation exposure. Based on the 252Cf radiation experiment results, we found that our SRDAS instrument can be used in the neutron monitoring and dose measurement in aircraft altitude. By the flight measurement, verified that our SRDAS instrument could be used as a reasonable monitor for space radiation dosimeter at aviation altitude. It should be noted that the Liulin instrument showed over-response by about 30% at aviation altitude than TEPC type equipment. These successive experiments showed that our SRDAS instrument can be practically utilized to measure in-situ aviation radiation.