1. INTRODUCTION
Active galactic nuclei are compact regions where enormous amounts of energy are released within a relatively small volume of space. Numerous compact relativistic objects in the form of a single (Hickox & Alexander 2018) and maybe even multiple (Gabányi et al. 2016) supermassive black holes with surrounding radiating gas-and-dust and stellar a aggregates have been detected in the centres of such regions to date. The study of such objects is challenging due to a wide variety of active galactic nuclei and the absence of unambiguous relationship between their properties. On this paper we will try to look for such distinctive features of these astrophysical systems that are associated with the manifestation of the motion of the positron component in the diffuse component of non-central (peripheral) parts of the active galactic nuclei.
Generation of high fluxes of fast particles (including atomic nuclei with high kinetic energies), as well as those of X-ray and γ-quanta, can be regarded as one of these common features. The leptonic component – namely, high-energy electrons and positrons, along with hard quanta - should be differentiated among the above indicated sources of excitation of the matter surrounding the galactic nucleus. Protons and atomic nuclei with the emitted alpha-particles have low penetrating power and cause active disintegration of molecules and dust in the central regions of the galactic nuclei. Spectroscopic feedback from these particles can hardly be observed due to shorter path lengths before stopping.
Calculations of the energy loss of electrons and positrons at very high and moderate energies in the interstellar medium were performed in many studies in the 1960-90s (Gould 1975;Gould 1990). The referenced authors detected a wide range of energies radiated by these leptons. These properties of cosmic rays have been factored in studying spectroscopic manifestations of active galactic nuclei. In particular, the maximum of the distribution of energy from off-center (peripheral) areas lies in the region of the hard ultraviolet and soft X-ray spectrum with a continuous component attributed to low-energy bremsstrahlung (braking) radiation and a line component produced via cascade transitions to vacant atomic K-L energy levels created due to the energy loss of fast leptons by ionisation.
The radiative dynamics of gas in active nuclei is not discussed in this study; it will be the subject of the next paper. We suppose that, as is the case with planetary nebula models, in galactic nuclei there is the transfer of X-rays and γ-rays from the compact source while the diffuse component of the radiation field is associated with the energy loss of fast leptons. In this case, the study of radiating surfaces of active galactic nuclei is a specific physical problem with set out parameters of the medium through which hard cosmic rays pass through.
In the subsequent sections, we will consider spectroscopic feedback from gas-and-dust aggregates for fast electrons and positrons and, separately, for the X-ray and γ-ray fluxes. For each case the total radiation field distribution functions S(E) and electron energy distribution functions F(E) are given. Spectral fluxes will be calculated using the Osterbrock-Lucy equations (Osterbrock & Ferland 2005;Doikov et al. 2018a, b). By spectroscopic response, we mean the radiation of a diffuse medium associated with the loss of energy by fast leptons through the ionization losses and the formation of K-vacancies in target atoms of the diffuse medium. This physical mechanism is common in the formation of hard ultraviolet and soft X-ray spectra.
Thus, of particular interest are the physical effects that manifest themselves in all regions of the AGN, which show the mechanisms of transportation and processing of energy from the center to the periphery of the AGN. The most probable and effective are cosmic rays. Their propagation occurs both along the disk of active galaxies and through their halos. If the energy of heavy cosmic rays reaches several GeV, we can expect the formation of a high-energy lepton component. The part of positrons is near 75% of all other leptons involved in the Δ-resonance.
Such a phenomenon can be the result of the interaction of positron fluxes with very cold gas-dust aggregates in the off-center part of the AGN. It should be emphasized that the nature of the interaction of positrons with matter varies depending on the state of aggregation. In gases, consisting of atoms and molecules, the processes of ionization losses of positrons and their annihilation are important. It is especially important to relate the physical conditions at the site of the interaction of positrons with target atoms and molecules, leading to annihilation. In particular, to identify the chemical elements that have experienced annihilation.
In the study by Doikov et al. (2017) and later investigations, the correlation between the morphology of the total emerging γ-ray flux and chemical composition of the medium in young supernova remnants was established. In the present paper, we consider positron fluxes in active galactic nuclei with a wider energy spectrum.
On the other hand, the existence of high positron fluxes itself raises a question about their origin. In young supernova remnants, these fluxes are generated through a chain of radioactive decay of the titanium isotope (Grebenev et al. 2012;Doikov & Andrievskii 2017). Let us be guided by models in which in the active galactic nuclei, which are the regions in the immediate vicinity of compact objects, powerful streams of high-energy particles and quanta are formed. Following the various routes along both disk and halo, these streams penetrate the peripheral parts of AGN. The positron component of incident stream is mixed with the positrons produced as a result of Δ-resonance. The energy distribution function of positron’s energy in this case has a maximum in the region of tens of MeV.
In the further sections, we will show the significance and availability of positron annihilation spectroscopy for observations based on the calculations of cross-sections for interactions between low-energy positrons and atoms in active galactic nuclei. We believe that regions in which secondary particles are formed in the collisions of protons, ultra-high-energy α-particles and atomic nuclei with hydrogen atoms as per the pattern () play a specific role in active galactic nuclei (Dermer 1984, 1986a, 1986b). In these regions, secondary positrons that contribute to the low-energy portion of the energy distribution function are produced, and these are the positrons that lay the foundation for positron annihilation spectroscopy. Besides secondary positrons and electrons, all other particles are rapidly thermalised within compact regions of active galactic nuclei.
Aiming at elaborating the classical concept on rare light elements (Lang 1974, Hayakawa 1969), the results of calculations of the single-photon annihilation of positrons with K-shell electrons of rare nuclei produced through spallation reactions will be presented in this paper. These atoms include the following elements: . The detected γ-radiation produced by the proton by excess nuclei can be associated with the additional Doppler broadening of their optical lines. This is because in a cold, rarefied diffuse medium, the width of the emission lines in the optical wavelength range is close to the natural one. Against this background, the presence of a recoil impulse in the nucleus as a result of nuclear transformations will produce a well detectable differences. The detected γ-ray emission may be related to the additional Doppler broadening of spectral lines of these rare elements.
Copious energy fluxes of all cosmic rays enable us to conclude that these elements are distributed throughout the entire bulk of active galactic nuclei, but they cannot be observed properly with conventional methods since the conditions of excitation of their spectral lines are not met.
Consequently, some optical transitions can be triggered (Doikov 2018a). The only supplementary source of information in this case is annihilation of cosmic ray positrons with emission of one or two photons. The role of other antiparticles is beyond the scope of this paper though cosmic showers detected in the upper layers of Earth’s atmosphere are indicative of their presence.
Main processes resulting in spallation reactions involve positrons and α-particles and do not produce any strong radiative feedback. In some cases, such nuclear reactions are accompanied by γ-quanta emission. Currently, due to the low yield of γ-quanta the description of these reactions is of no practical interest within the problem addressed in this paper. The energy loss of ultra-high-energy particles (with energies over 30 MeV) results in the emission of γ-quanta contributing to the diffuse component. It is only γ-quanta emitted in the one-photon annihilation that are able to produce a broadening of lines, and it will be proved by calculations in this study.
2. THE IMPACT OF SOLID-STATE GRAINS ON THE GAS-AND-DUST AGGREGATES
Fission fragments and fluxes of high-energy nuclei, i.e. heavy cosmic rays, formed in immediate proximity to massive black holes, play an important role in inner regions of active galactic nuclei. They are known for their low penetrating power and, consequently, high ionisation efficiency. Characteristic radiation associated with the interaction of these fluxes with gas-and-dust aggregations should be detected in order to adequately observe inner regions of active galactic nuclei. Evolution of galactic nuclei is not related to active star formation or supernovae; thus, because the spallation reactions caused by massive component of cosmic rays are smaller in the regions where these fluxes penetrate AGN’s diffuse media the meaningful abundances of heavy elements cannot be expected. Meanwhile, the primary fluxes of heavy nuclei may be represented by an assembly of protons and α-particles within a wide range of high energies. The impact of electron and positron components will be discussed in the next section.
The interaction between protons and α-particles with matter has been the subject of numerous studies. However, there is still a heightened interest in the problems on standardisation of radiative feedback from the fluxes of mentioned particles propagating through gas and dust. The computer code for the calculation of dynamical evolution of galactic cosmic rays was developed by Moskalenko & Strong (1998). Thus, today, having known parameters of particles and target media, it is feasible to calculate the flux of cosmic rays arriving at the observer’s detector.
As regards the problem on the radiative feedback generated by a gas-and-dust aggregate, let us consider separately the impact of the fluxes of these particles on the gas and dust. As is the case in the well-studied showers of secondary particles produced by protons and α-particles, the final-state particles in showers are high-energy electrons, positrons and muons. Hence, the luminescence of the upper layers of active AGN is associated with the interaction of the final-state products of such showers with hard X-ray and γ-ray quanta that reach these atmospheric layers.
In this section, we will discuss the determination of the radiation field structure and particle energy distribution throughout elementary volume of the surface matter in active galactic nuclei. A similar problem for supernova remnants has been already solved for the calibrated flux of positrons in the young remnant of supernova SN 1987A and yielded initial functions S(E) and f(E) (Doikov et al. 2018a; see Figs. 1-3). Then, we will obtain the total contribution of protons, α-particles, electrons, positrons and muons in order to calculate S(E) and f(E).
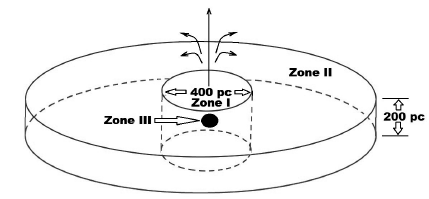
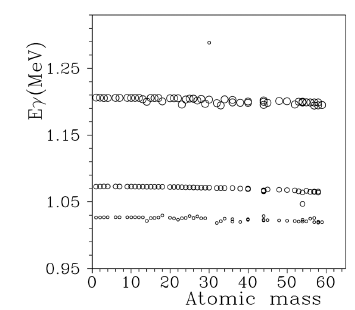
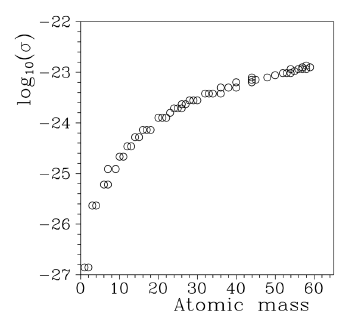
3. PROTONS AND Α-PARTICLES
The classical Bethe-Bloch formula for the linear loss of kinetic energy T due to ionisation along the X axis holds for high-energy protons and α-particles. The formula written in the most suitable manner is as follows:
Here R is the free path of particles; v1, m and Z’ are the velocity, total relativistic dynamic mass and charge of the incident proton or α-particle, respectively; M, v2 and Z are the mass, velocity and charge of target atoms, respectively; N is the current number of target atoms involved into interaction with the afore-mentioned incident particles. Producing a radiative feedback requires that de Broglie wavelength be comparable to the scales of K-shells of target atoms and that heavy cosmic rays eject electrons from the innermost shells. Such energies are attributed to incident particles in the middle to outer regions of active galactic nuclei.
This assumption is true provided that the mentioned particles do not participate in the radioactive transformations, i.e. they are not secondary particles. Particles that reach the surface of active galactic nucleus are predominantly secondary and no longer have relativistic kinetic energies in Earth’s atmosphere. In this case, we expect to detect recombination lines of double-ionised hydrogen and helium. In other words, active galactic nuclei, through which protons and α-particles pass, exhibit overionisation in compact regions, though major portion of their energy is used in the spallation reactions.
According to the classical theory of atomic collisions developed by Mott and Massey, the most efficient energy transfer is observed at comparable masses of colliding particles (see Eq. 1 in Doikov et al. 2018a). It means that protons and α-particles lose their kinetic energies 103 times more efficiently than electrons and positrons, and therefore they are mainly secondary particles. Given the conserved kinetic energy, primary protons and α-particles heat the inner regions of active galactic nuclei more efficiently as compared to other high-energy particles.
These conclusions are fair provided the absolute sphericity of AGN observed along their projection plane. A disc-shaped distribution of matter in the projection plane is quite often the case. The main portion of the energy of hard quanta and fast particles is concentrated in directions close to the normal. Jets are observed in the same directions. This is why such outer regions are subjected to their combined impact. In this case, we refer to the ionisation loss as the energy loss due to the bremsstrahlung emission, electron-positron pair production and ordinary Coulomb interactions with ejection of K-L-shell electrons.
The X-ray and to a lesser extent γ-ray emission generated in directions close to the normal is associated with bremsstrahlung rather than cascade transitions in metal atoms. The presence of well-measured continuous emission fluxes resulted from the motion of relativistic particles at the short-frequency end of the spectrum, which spans hard ultraviolet to γ-rays bands, and their separation from the Compton emission enables us to distinguish a spectral component directed towards the galactic discs. It is important for any location of galaxies relative to a terrestrial observer.
High-energy cosmic particles captured and accelerated by galactic magnetic fields are ejected strictly along the normal towards the galactic projection plane and detected in the near-Earth space. Unlike fast electrons and positrons, protons and α-particles do not directly cause the bremsstrahlung radiation for which the cross-section is either many orders of magnitude smaller or absolutely forbidden by the laws of quantum mechanics. Synchrotron emission of these cosmic rays is lower by an order of the mass ratio.
4. SPECTRAL DIAGNOSTICS DIRECTED PARALLEL TO AN OBSERVER’S LINE OF SIGHT
The activity in galactic nuclei is an important aspect in evolution of galaxies of different types. Galaxies hosting active galactic nuclei in different planes with respect to a terrestrial observer have been observed. In most cases, observations detect integrated characteristics of active galactic nuclei. Hence, the selection of homologically similar galaxies with AGN in different projection planes is essential for their studying.
As regards the experience of obtaining spectroscopic data gained in research of young supernova remnants, the outer regions of AGN which belong to the relevant galactic discs are affected by high-energy fluxes of particles and quanta of extreme low energies (0.1-10 MeV); in particular, the majority of these particles and quanta have energies not exceeding 1 MeV. As has been shown in the studies by Doikov et al. (2017;2018a,b), the secondary electrons and positrons of such energies effectively interact with K-L shell electrons of almost all atoms in the near-surface layers of AGN.
Gas-and-dust aggregates associated with active galactic nuclei serve as an important component in the course of their evolution. At the early stages of formation of AGN, gradual disintegration of some portion of molecules and dust in the inner regions occurs with the remainder being simultaneously pushed out towards the periphery of the active galactic nucleus. Unlike young supernova remnants where monochromatic flux of fast positrons serves as the source of excitation of atoms and molecules, as well as the source of the dust heating, the outer region of AGN are affected by secondary electrons, positrons and short-wave quanta with a wide range of energies. During such separation of matter, the local area of the nucleus is being enriched by metals, silicon and CNO elements composing the dust grains; hence, high-power diffuse radiation is generated in the outer regions of active galactic nuclei.
In accordance with the monograph of Osterbrock (1989), we describe the emerging radiation flux Η(τν) associated with the presence of dust. The relations obtained by Osterbrock were modified by Lucy (1991). The resulting equations are suitable for interpreting infrared fluxes from active galactic nuclei; however, for several reasons they cannot be employed for the short-wavelength portion of the spectrum. Non-thermal nature of the radiation field should be factored in to solve the equation of radiative transfer.
On the other hand, the electron energy distribution function takes a specific form similar to that one observed in young supernova remnants (Doikov et al. 2018a,b). Spectral features of surfaces in active galactic nuclei radiating towards the galactic discs at short wavelengths are defined by a series of cascade transitions to the created K-L vacancies, as well as by high-energy Auger electrons.
In this study, we perform direct calculations of the local distribution of quanta energy Е, the source function S(E) and the function of Auger electron energy distribution f (E) in the near-surface layers in active galactic nuclei. Initially, the calculations are performed given the presence of K-L vacant energy levels in atoms resulted from the energy loss of fast particles due to ionisation. The calculations of quanta state with core holes in the afore-specified energy levels show that the highest energy is emitted in quanta when a K-vacancy is present. When fast particles interact with multi-electron atoms, simultaneous creation of the K and L vacancies or two holes at the K energy levels is not impossible.
In this case, the motion of outer electrons towards the K-vacancy breaks the order of cascade transitions and yields emission of specific quanta. Besides, the sequence of quanta emission changes – namely, a low-energy quantum is emitted first, followed by a high-energy one. If it is not forbidden by the selection rules for the energy levels, an outer electron can also hit the K-shell straightaway. Positrons and electrons within a rather wide range of relativistic kinetic energies affect target atoms practically in a similar manner. When positrons reach kinetic energies comparable to the kinetic energies of the K-L-shell electrons, their annihilation occurs. Spectroscopic consequences of this process consist in emitting γ-quanta at the accurately determined frequency, which is defined by the laws of conservation of energy, momentum and charge in a closed physical system.
Depending on the binding energy of a K-L-shell electron, the corresponding γ-quantum is emitted at its own specific frequency, which is shifted relative to the frequencies of γ-quanta emitted by other elements. The value of such a frequency shift is important for identification of spectral lines of the elements, which are of interest to us. In this regard, positron annihilation spectroscopy is inherently a high-precision technique. At high intensities of positron fluxes, which are expected in active galactic nuclei, the intensities of quanta fluxes in the sought γ-lines will noticeably, by several orders of magnitude, differ from the corresponding intensities in the continuous spectrum.
As it was noted above, the γ-quanta continuous spectrum is produced by particles of very high energies as a result of the direct and inverse Compton effect, as well as bremsstrahlung, at moderate kinetic energies of cosmic rays. These issues will be the subject of a separate paper.
In contrast to young supernova remnants, in active galactic nuclei (AGN) it is impossible to unambiguously detect the sources of excitations of atoms by fast particles; such calculations would be purely academic given the absence of positron annihilation in the K energy levels. Annihilation of positrons with free electrons is less likely due to extremely low density of interstellar medium. If the medium in the central regions of AGN is metal-deficient, it accounts for even lower density of electrons as compared to that in the interstellar medium. Taking into consideration strong fluxes of high-energy particles in active galactic nuclei, the formation of rare-Earth elements in the spallation reactions is expected.
This phenomenon should be investigated in the innermost regions of AGN and in the entire bulk of the surrounding halo. In this case, it is worth calculating precise energies (frequencies) of γ-quanta for all rare-Earth elements. In so doing, we will take into account only those elements, which can be supplied to the interstellar medium as a result of the destruction of dust grains.
5. BASIC ASPECTS OF THE γ-INDUCED POSITRON ANNIHILATION SPECTROSCOPY OF AGN
Gamma-induced positron annihilation spectroscopy is based on the usage of special detectors for quanta with frequencies of 1018 – 1022 Hz. An important aspect in planning observations is the spectroscopic data on the γ-lines valuable for the addressed problem of identification of elements. To this end, we are to solve the set problem on calculation of energy (frequency) for the following elements: Н, Не, С, N, O, Be, Mg, Ca, Sr, Ba, and Si. The selection of elements is based on the following criteria: Н and Не are the relict elements; C, N, O, Si, and Mg are elements resulted from the destruction of dust grains in the interstellar medium; Be, Mg, Ca, Sr, Ba, and Si are produced in the spallation reactions through exposure to high fluxes of cosmic rays.
Such a selection of elements is only provisional; their list will be extended in the course of observations. Positrons have rather high energies in the inner regions of active galactic nuclei, and their annihilation with bound electrons only occurs after a considerable number of collisions. The pattern of interactions between incident positrons and the afore-mentioned atoms is practically the same.
Mikhailov et al. (2013), who summarized theoretical and experimental studies in this field, mainly reports the cross-sections for the interaction of positrons with different atoms of Mendeleev’s periodic table of elements.
An important conclusion made in the above-referenced paper is that the one-photon annihilation with one K-electron accompanied by ejecting another K-electron is very likely. As noted previously, it means that the energy of the emitted γ-quanta depends on the binding energy of the electron removed via the one-photon annihilation channel. The target atom itself enters a highly excited state with no K-electrons at all, i.e. undergoes sequential double ionisation. The one-photon annihilation channels can be presented as follows:
Both processes can be observed for all elements from helium onwards. In cold gas-and-dust aggregates, both processes are of interest with regard to spectroscopic determinations of abundances of elements and their isotope separation. At nonrelativistic energies of positrons, the cross-section for double ionisation (corresponding to the second channel in Eq. (2) may exceed the cross-section for single ionisation for light elements.
The calculation of positron annihilation processes in different atoms is additionally simplified, since the wave functions of the K--hell electrons are similar to those of the helium atom. It means that they are not shielded and do not require calculations of electron oscillations. The first channel in Eq. (2) is responsible for the transfer of the highest momentum to the nucleus. It means that the nuclei gain appreciable kinetic energies just like daughter nuclei do in nuclear decays.
The ratio of the first and second cross-sections ionisation channels in the single-photon annihilation of positrons with K-shell electrons is true for the low-energy portion of positrons (the so-called low end of the positron energy spectrum): , where Z is the atomic number of an element and B = 0.090.
The probability of double ionisation is much higher for light elements. In this case, the main portion of energy is carried away by the emitted γ-quantum. Differentiation of energies of the emerging γ-quanta is a valuable aspect in positron annihilation spectroscopy. In case of double ionisation in the course of the single-photon annihilation, the energy is distributed between the recoiling nucleus, ejected K-shell electron and emitted γ-quantum. The recoiling nucleus energy in such a process is negligible; hence, the energies of the emitted γ-quanta will differ by the binding energy of the respective K-shell electrons. For the above-mentioned light elements, this difference is tens of eV; for the iron-group elements it reaches hundreds of eV. Thus, this factor is a crucial source of information for the chemical analysis of the supernova remnants and AGN. Fast positrons tend to cause the energy loss due to ionisation in matter.
At ultrarelativistic energies, electrons and positrons provoke bremsstrahlung and continuous Compton scattering in the field of atomic nuclei which is beyond the scope of phenomena discussed in this paper (Gould 1990). In the high-energy end of the spectrum, there are a continuous component and lines produced in annihilation of low-energy positrons. The X-ray and ultraviolet lines yielded by cascade transitions will also combine and overlap the continuous emission resulted from scattering of the afore-described types. As it was reported in Doikov et al. (2018a), positrons lose the major portion of their kinetic energy due to ionisation in the discs of AGN and participate in the formation of the radiation field of active galactic nuclei on the same basis as other cosmic rays. It is not until they decelerate, that we are able to identify the chemical composition of matter.
It is more convenient to carry out the isotope separation of chemical elements in atoms or molecules at the instant of positron annihilation with a K-shell electron for the groups of atoms with maximum energies of recoiling nuclei. This applies to light elements. In the processes discussed above, it is suitable to observe such an event in the course of double ionisation of atoms and molecules in cases when the nuclei of free atoms or atoms included into molecules gain maximum recoil energy while the annihilation occurs with emission of a single photon. It has been reported in some experimental studies that in such cases the recoiling nucleus energy can be efficiently increased at the expense of a decrease in the momentum of the injected second K-shell electron and, to a lesser extent, a change in the γ-quantum momentum.
Even in this case, the momentum of the γ-quantum is very sensitive to changes in its kinetic energy. Similar features can be observed in radioactive molecules when the momentum of the neutrino emitted upon the K-capture is not adequate to destroy the parent molecule (Doikov & Andrievskii 2017). Thus, when describing triple collisions as given in (3) in non-relativistic approximation, the maximum cross-sections for the processes defined by (2) and (3) can be achieved when examining the interaction of a non-relativistic positron with the first K-shell electron in the nuclear field:
At the first stage, a positron and a K-shell electron form a positron in the same nuclear field, which is followed by its decay with creation of two K-vacancies. The first vacant energy level is the result of annihilation of the positron and K-shell electron while the second one is created upon the ejection of the second K-shell electron after transfer of a portion of the energy of annihilating particles, i.e. in the so-called K-Auger process. Despite the fact that secondary K-shell electrons are slow, the energy of the resulting γ-quantum and its frequency are quite sensitive to the significant momentum transferred to the nucleus. Thus, the total energy of this process can be estimated as the balance of the kinetic energy of the recoiling nucleus En and γ-quantum reduced by the binding energy of the K-shell electron Eb:
With respect to spectroscopic measurements in γ-ray spectra, this result allows the identification and recognition of the isotope shift by recording γ-quanta shifted in frequency. The problems related to the determination of values included into Eq. (4) can be partially solved by calculations of Eb in the Hartree-Fock approximation and they are well known in tabular form. The energy of recoiling nuclei of different elements can be easily determined experimentally, since the initial kinetic energy (velocity) of the incident-annihilating positron is known. In cold supernova remnants and regions of active galactic nuclei close to the central compact source, the recoiling nuclei with appreciable kinetic energies may only appear as a result of nuclear transformations.
Their presence in the non-thermal cold gas-and-dust plasma results in the presence of doubly charged ions, i.e. excessive non-thermal ionisation. For instance, as described for the supernova remnants in Doikov et al. (2017;2018a), the final products of the radioactive decays in the supernova remnants only exist in an ionised state. As an example, in the above-referenced study, the ion that is the final product of the decay chain was considered; its spectra were quite easily identified in the optical spectra of the supernova SN 1987A remnant with the Hubble Space Telescope on 07 January 1995, as late as eight years after the supernova explosion. In isotopes of light nuclei the binding energy Eb is not sensitive to their atomic mass.
These methods are quite applicable for investigations in young diffuse supernova remnants and outer regions of active galactic nuclei. To plot the emerging spectrum of the specified objects at the γ-quanta frequencies, let us write down the equation for the optical depth formed within a given energy range:
The estimated abundances of various elements are presented as average values for young supernova remnants; the same is true for the scales of the investigated objects. The outer regions of AGN and young supernova remnants are identical in their physical state. In case of active galactic nuclei, the second channel of Eq. (2) should also be factored in. High fluxes of diffusely distributed positrons in young supernova remnants are produced in a series of radioactive decays of the titanium isotope . Energies of positrons are distributed isotropically within the range from hundreds eV to several MeV peaking at 0.5-0.6 MeV. The ratio between and is inversely proportional to the atomic number. Hence, we adopt the cross-sections estimated in the study by Mikhailov et al. (2013 and reference therein) to calculate the optical depth using Eq. (4).
In order to perform mapping of young supernova remnants, Osterbrock (1989), Osterbrock & Ferland (2005) and Lucy (1991) suggested the Osterbrock-Lucy equation of radiative transfer for volumetrically distributed emitters.
Since this equation implies dust grains as emitters, we have to adjust it to factor in the propagation of γ-quanta in the supernova remnants and outer regions of AGN close to the disc component.
Given the absence of a central source of radiation, we will use the Osterbrock-Lucy equation for the emitters and absorbers uniformly distributed throughout the radiating bulk:
where . In the γ-ray region, three processes contribute to the total emerging flux, namely the γ-quanta emission induced by positrons, their absorption in matter and Compton scattering with atomic K-shell electron in the nuclear field . At the investigated energies, the value ω is sufficiently small due to insignificant Compton scattering - ω≈0.01 - which is not true for the inner regions of active galactic nuclei. The presence of the graphite dust and astronomical silicates in these objects change the ratio between scattering and absorption of γ-quanta towards the increased ω. However, this aspect is beyond the scope of this study.
Given that x is the γ-ray emission per unit volume of a clump and can be calculated by Eqs. (6)-(7), and employing the Osterbrock-Lucy equation adjusted by Varosi & Dwek (1999), we obtain equations which are suitable for calculations the intensity and total emerging flux :
For the clumps with different composition, the total emerging flux depends on the ratio between the scattering, absorption and emission coefficients. The structure of the radiation field of clumps containing no dust was described by Doikov et al. (2018a,b). Namely, the source function S(E,r) was defined. The presence of a substantial amount of the graphite dust in the ejecta should have noticeably suppressed the short-wavelength portion of the source function S(E,r) that could have made the production of the remnant atomic spectra, de facto observed in 1995, impossible. For the same reason, the luminescence and availability of young supernova remnants and outer regions of active galactic nuclei for investigation enables us to deduce that graphite is already converted into gaseous state through evaporation while astronomical silicates and other metal-containing particles are in the condensed phase.
Inner regions of AGN are close to the emitters of high-energy cosmic rays (Fabian 2012;Moskalenko & Strong 1998). The energy loss of all components of cosmic rays due to ionisation occurs at energies below 30 MeV (Gould 1990). The main goal of the observational astronomy is to determine the energy distribution for high-energy quanta and particles in the central regions of AGN associated with massive black holes. Unlike black holes of stellar origin, a black hole in AGN nucleus has angular momentum, which is noticeably smaller; hence, its gaining high angular velocity can hardly be expected. Fig. 1 shows the schematic view of inner regions of AGN, discussed here after.
Electrons, positrons, protons and α-particles can acquire ultra-relativistic velocities either as a result of abnormally strong explosions when ambient matter falls upon the compact component or due to the presence of a magnetic field in this compact object.
The present study aims at solving the problem of spectroscopic diagnostics of matter under conditions typical for the central regions of active galactic nuclei. Combined processes in ultra-relativistic plasma, as well as problems on stellar dynamics, will not be considered in this paper. Correlated active observations of active galactic nuclei in the submillimetre and X-ray regions allowed the estimation of the lower limit of the formed bolometric flux with energy Fth ≈ 1062 erg. The active phase of galactic nuclei is assumed to be about 108 years, which is associated with the depletion in gas and dust reserves in the regions surrounding the galactic nuclei. According to the estimates by Ferland et al. (1998), the flux of cosmic rays should be proportional to the filament luminosity and equal to the entire investigated bulk average (solar masses per year):
In these regions, cosmic rays when colliding with cold gas-and-dust aggregates, heat the filaments and produce secondary particles with energies up to 40 eV. Secondary particles, in turn, heat the gas and excite atoms and molecules. The adiabatic exponent of relativistic gas in the surroundings of the black hole is γ=4/3.
The X-ray transitions of fully ionised atoms of Fe XXVIII (15 Å and 17 Å), O VIII (19 Å) and O VII (22 Å) were observed in the inner regions with the Chandra X-ray Observatory. The preliminary estimate of the mechanical energy transferred to cosmic rays is Hmech = 1045erg∙s-1. Without loss of generality, we assume that the area of complete thermalisation in AGN is 400 pc. As is the case with the cosmic rays detected in the upper layers of Earth’s atmosphere, the presence of fluxes of high-energy protons and α-particles results in the creation of proton-rich nuclei upon the collision with light elements (in our case, including CNO elements) and generation of the secondary positron flux.
Such a process is energetically beneficial in the inner regions of AGN and can be extended up to 300 pc. As noted earlier, given the presence of a significant angular momentum around the central compact source, the positron annihilation areas will produce a line γ-ray spectrum against the background of continuous Compton scattering and bremsstrahlung radiation if the line of sight of an observer is at angles close to the normal to the active galactic discs. Emission of high-energy gamma-rays is also capable to produce electron-positron pairs in the nuclear field. Moreover, positrons which are the closest to black holes are generated at the event horizon.
Thus, high-energy positrons with high penetrating power affect the majority of active galactic nuclei. Annihilation of these positrons after their decelerating in the layer of 300 pc thick will produce γ-quanta of strictly determined frequencies depending on the atomic number of the chemical element. In other words, it will define the chemical composition of the bulk of active galactic nuclei.
Summarizing this section, we would like to note that despite the eruptive processes in the central regions of AGN, the directed fluxes of positrons eventually mix with secondary positrons produced in collisions with light nuclei and form a function of their diffusely distributed kinetic energies. This fact makes it possible to look at any region of AGN with regard to spectroscopic identification of elemental abundances. When describing the one-photon annihilation, it is necessary to take into account that annihilation with a K-shell electron is the most probable for those positrons, which have kinetic energies comparable to the kinetic energy of the K-electron.
For the first channel of the single-photon annihilation, the set of equations of conservation of energy and momentum can be written as follows:
By adopting the results of the study by Mikhailov et al. (1989), we obtain the set of equations:
The values given in bold are vectors. To numerically solving this system of equations, it is necessary to square the second equation, thus yielding a new variable, which is the angle between the recoiling nucleus momentum and γ-quantum momentum. Let us denote this angle as α. Then, the second equation from the set of Eq. (12) will be written as follows:
As reported by Doikov et al. (2018a), when the energies of incident positrons are close to the binding energy of the K-shell electron or exceed it, the emission angle α between the atom and γ-quantum takes the value within the range 0≪α≪0.01 rad. Averaging the second equation by angles α yields the average cosine of the emission angle cosα≈1. This result is associated with the isotropic (diffuse) motion of positrons in the supernova remnants and the bulk of AGN. We will tackle the set problem in the final form by numerical solving the set of equations presented below:
The results of calculations of Eγ depending on the atomic mass are given in Fig. 2. The correlations coefficients show the slow decrease of Eγ with the increase of atomic mass for fixed initial positron kinetic energies. The main result is the increase of Eγ with the increase of initial positron kinetic energy. It is worth to point that the observed energies of γ-lines allow the estimation of positron kinetic energies, and the result is not strongly dependent on the atomic mass.
Fig. 3 is the dependence of one-photon annihilation cross-section against the atomic masses for positron energies similar to the binding energies of K-electrons. These cross sections allow the discussion of annihilation probabilities in the given point of AGN. The initial energies of secondary positrons can be in the range from 1 to 20 MeV. Taking into account the supposed diffusion distribution of these positrons, it is possible to expect the loss of their energies due to braking and also one-photon annihilation.
The following can be deduced from the results obtained:
For all given elements, the energy (frequency) of the γ-quantum emitted upon the one-photon annihilation depends significantly on the characteristics of atoms;
The energies of γ-quanta given in Fig. 2 can be measured using ground-based and space instruments;
At the low-end of the energy spectrum of the positron flux an alternative production of γ-quanta via the pattern described in this section is allowed; in other words, γ-quanta may also be generated in the annihilation of positrons with incident K-electrons rather than in intranuclear radiative transitions;
The structure of γ-ray spectral lines of active galactic nuclei is governed by the γ-quanta emission by each atom;
Unlike young supernova remnants where conditions are not stationary and the physical parameters change regularly, in the used model it is believed that bulk of AGN are in are relatively stationary state. That is, the considered particles of cosmic rays collide with fixed targets as it was described here before, but the collective movement of diffuse component within the space occupied by AGN is insignificant.
5. CONCLUSIONS AND DISCUSSION OF THE RESULTS
We have suggested new or adjusted the existing ratios suitable for spectroscopic diagnostics of media like young supernova remnants and AGN with similar morphological properties. The knowledge about chemical composition of such media and their isotopic composition enables us to draw conclusions about asymmetrical geometry of supernova explosions, their chemical composition and evolution in a way, which is independent on the existing methods.
The fundamental aspect of the introduced new method is that even if the arbitrary positron energy (velocity) distribution function F(E) or F(v) is present, the radiative feedback of the medium does not depend on F(E). Formation of positron in the energy levels occupied by atomic K-electrons requires the energy characteristics and, consequently, kinematics of the incident positron to be comparable with those of these K-shell electrons. If this is the case, the one-photon annihilation occurs with emission of γ-quantum of strictly defined frequency corresponding to an isotope of this element. In young supernova remnants, a flux of monoenergetic positrons is produced in the decay chain . After a thousand of collisions each of positrons annihilates with a K-shell electron of atoms in the surrounding medium.
The given list of elements present in such remnants also provides data on their isotopes of different origin. In AGN, positrons have a wider range of energies, higher mobility and penetrating power. Prior to generation of the sought γ-quantum, a positron should undergo from 106 to 107 collisions. In this case, the maximum path length of the positron before stopping is 300 pc. It means that with means of positron annihilation spectroscopy we can cover the whole area occupied by active galactic nuclei.
By determining the parameters included in Eq. (8)-(10), we can plot a three-dimensional image of the supernova SN 1987A remnant and standard active galactic nucleus with parameters specified in the previous section. One of the valuable characteristics of the ongoing important nuclear processes is the isotopic composition of atoms available for observations. For instance, the calcium isotopes and have different origin and are suitable for observations in the optical band. But the ratio of these isotopes could only be estimated from the high-dispersion molecular spectra. In so doing, positron annihilation spectroscopy allows of the high-precision separation of these isotopes, which extends the range of the investigated objects for which the isotope ratio is defined by other methods.
In the present study, we have also considered the impact of heavier particles, such as protons and α-particles. According to the estimates reported by Osterbrock & Ferland (2005), these particles do not directly influence thermodynamic and radiation features of the inner regions of AGN. However, these particles do not exhibit any characteristic radiation features, which could be used to determine the chemical composition. The most important effect of their presence is the ongoing spallation with formation of rare-Earth elements. Spallation reactions yield positively charged isotopes of light elements, some of them are unstable and prone to positron emission.
According to the results of the studies by Gould (1990) and Mikhailov et al. (1998, 2013), the one-photon annihilation of positrons with high Lorenz factor is less likely to occur (its cross-section is smaller by three orders of magnitude) as compared to the energy loss due to ionisation. This is why even in AGN it is only the low-energy end of the positrons energy distribution function, which should be taken into account.
Positron annihilation spectroscopy of cold gas-and-dust aggregations makes possible the following:
To determine the percentage of gas and dust in both interstellar matter and in young supernova remnants;
To identify chemical elements by analysing the single-photon annihilation of positrons with atoms;
To find the contribution of the low-energy component of the positron flux at the sites of γ-radiation from the calculated γ-ray fluxes;
To detect regions in AGN which have not previously been accessible for quantitative analysis;
To distinguish regions of ongoing intense nucleosynthesis in active galactic nuclei and in their surroundings.
Spectroscopic effects of the transfer of a portion of the total colliding system energy En, calculated by the set of Eq. (14), to a nucleus have not been taken into account in this study. It will be the subject of a separate paper. However, it can be preliminary deduced that the lightest nuclei tend to have the highest velocities. These velocities markedly exceed the corresponding thermal velocities. In this case, the annihilation of a positron with a K-shell electron correlates with the presence of recoiling atoms with non-thermal velocities and results in a noticeable symmetrical Doppler broadening of the atomic lines in the nebular component of AGN.
If we have intensive flux of secondary positrons in the diffuse gas and dust environment then the positron’s braking due to Δ-resonance allows to estimate the separation of condensed and gaseous components using the observed intensity ratios of one-photon and two-photon annihilation lines. The observations of COMTEL, INTEGRAL and other missions are low sensitive for energies close to 1.022 MeV. That is why the one-photon annihilation part of the spectrum requires additional observations.
In this paper, we propose the way to estimate the level of one-photon annihilation. We found the relation between the recoil energy of nucleus En, the atomic number of chemical element and the Doppler broadening of the lines in the optical and infrared spectrum. We can conclude the possible observation of significant broadening for atomic spectral lines formed in the diffuse environment, where it is usual to expect only the natural width of spectral lines.